Org. Synth. 2007, 84, 306
DOI: 10.15227/orgsyn.084.0306
CHIRAL LITHIUM AMIDE BASE DESYMMETRIZATION OF A RING FUSED IMIDE: FORMATION OF (3aS,7aS)-2-[2-(3,4-DIMETHOXYPHENYL)-ETHYL]-1,3-DIOXO-OCTAHYDRO-ISOINDOLE-3a-CARBOXYLIC ACID METHYL ESTER
Submitted by Vincent Rodeschini, Nigel S. Simpkins, and Fengzhi Zhang
1.
Checked by Melissa A. Beenen and Jonathan A. Ellman.
1. Procedure
A. 1(S),2(S)-Diphenyl-N,N'-bis-[1(R)-phenyl-ethyl]-ethane-1,2-diamine (2). In a 500-mL, three-necked, round-bottomed flask equipped with a N2 inlet, two septa and a magnetic stirring bar is introduced successively CH2Cl2 (180 mL) (Note 1) and an aqueous glyoxal solution (9.40 mL, 82.5 mmol, 1.0 equiv) (Note 2) by syringe through one of the septa. One septum is temporarily removed and solid magnesium sulfate (40.0 g) (Note 3) is then added portion-wise over 20 min, while the mixture is stirred at room temperature. The resulting suspension is stirred for an additional 10 min, and then (R)-(+)-phenylethylamine (21.0 mL, 20.0 g, 165.0 mmol, 2.0 equiv) (Note 4) is introduced dropwise via syringe over a 5 min period. The resulting mixture is stirred overnight at room temperature. Magnesium sulfate is removed by filtration and rinsed with CH2Cl2 (2 × 25 mL). The filtrate is then concentrated by rotary evaporation and then further dried under high vacuum for 1 h (Note 5) to provide crude bis-imine (1) (Note 6) as an orange oil (21.3 g, 98.0%), which is used directly for the next step without further purification. Into a 1-L, three-necked, round-bottomed flask (Note 7) equipped with one low temperature thermometer, one N2 inlet and one septum is introduced crude bis-imine 1 (21.3 g) as a solution in Et2O (300 mL) by syringe (Note 8). This solution is cooled to −78 °C (dry ice-acetone bath), and PhMgCl (162 mL, 2.0 M in THF, 325 mmol, 4.0 equiv) (Note 9) is added drop-wise via syringe pump (Note 10) while maintaining the internal temperature between −78 °C and −75 °C over 2 h (Note 11). The resulting dark brown mixture is allowed to warm to room temperature gradually over 4 h, and stirred for an additional 2 h at room temperature. The mixture is then cooled to 4 °C (ice/water bath), and carefully quenched by the addition of a saturated aqueous NH4Cl solution (200 mL) after removal of the septum over 30 min. The solid that is formed is dissolved by the addition of de-ionized water (100 mL), and the two phases are separated in a 1-L separatory funnel. The aqueous phase is then further extracted with ethyl acetate (3 × 150 mL). The combined organic phases are washed with brine (50.0 mL), then dried over MgSO4 (30 g, 5 min) and filtered. The filtrate is concentrated by rotary evaporation and further dried under high vacuum for 1 h. The crude product (33.5 g) is purified by flash column chromatography. Thus, the crude brown solid is dissolved in the minimum amount of CH2Cl2 (approximately 10 mL), charged on a column (8 × 16 cm) containing 400 g of silica gel (Note 12) and eluted with Et2O-petroleum ether (5:95, 2 L, then 10:90, 2 L). After the first apolar impurity (this spot is only evident by UV), the title compound elutes, before a mixture of other isomers (Note 13). The combined fractions of the major isomer are concentrated by rotary evaporation to provide a slightly yellow solid (16.8 g). This solid is dissolved in boiling petroleum ether (250 mL), with addition of a small amount of CH2Cl2 (5 mL) to complete the dissolution. The solvent is allowed to evaporate to 1/5 of the initial volume at room temperature over 2 days, allowing the crystallisation to occur (Note 14). These crystals are collected by filtration, and rinsed with a small amount (15.0 mL) of cold (0 °C) petroleum ether. Thus, 13.8–14.7 g (40–43%) of pure diamine 2 are obtained (Note 15).
B. (3aS,7aR)-2-[2-(3,4-Dimethoxyphenyl)-ethyl]-hexahydro-isoindole-1,3-dione (3). Into a 250-mL, one-necked, round-bottomed flask, equipped with a septum and a magnetic stirring bar, containing cyclohexanedicarboxylic anhydride (8.50 g, 55.1 mmol, 1.0 equiv) (Note 16) is added glacial acetic acid (100 mL), followed by 2-(3,4-dimethoxyphenyl)ethylamine (10.1 g, 55.4 mmol, 1.0 equiv) (Note 17). The septum is removed and the flask is equipped with a Liebig condenser. The reaction is then heated at reflux (oil bath temperature 120 °C) for 13 h. The resulting mixture is allowed to cool to room temperature and poured into a 1-L beaker containing 400 mL of de-ionized water. The aqueous phase is extracted with diethyl ether (5 × 100 mL). The combined organic phases are transferred into a 1-L Erlenmeyer flask, cooled to 4 °C (water/ice bath) and neutralized by the slow addition (30 min) of a saturated aqueous Na2CO3 solution (150 mL) under vigorous stirring. After the addition is finished, the two phases are separated and the organic phase is further washed with saturated aqueous Na2CO3 (2 × 50 mL). The organic phase is then dried over anhydrous MgSO4 (20.0 g, 5 min). After removal of the solid by filtration, the organic phase is concentrated by rotary evaporation and further dried under high vacuum for 1 h. The resulting yellow solid is recrystallized from boiling light petroleum ether/ethyl acetate (100 mL:25 mL). Crystallization is allowed to occur at room temperature over 2 h, then at 4 °C overnight. The crystals are collected by filtration and washed with cold (0 °C) petroleum ether (25 mL) to give 14.2 g (81%) (Note 18) of the title compound 3 (Note 19).
C. (3aS,7aS)-2-[2-(3,4-Dimethoxyphenyl)-ethyl]-1,3-dioxo-octahydro-isoindole-3a-carboxylic acid methyl ester (5). Into a 100-mL, two-necked, round-bottomed flask (Note 7), equipped with one septum and one low temperature thermometer, containing a solution of diamine 2 (8.79 g, 20.9 mmol, 1.1 equiv) in THF (50 mL) (Note 20) cooled to −78 °C (dry ice-acetone bath) is introduced n-BuLi (8.50 mL, 2.5 M in hexanes, 21.3 mmol, 1.1 equiv) (Note 21) dropwise via syringe over 25 min maintaining the internal temperature between −78 °C and −75 °C. The resulting pink solution is allowed to warm to room temperature (23 °C) by removing the cooling bath, and then stirred at this temperature for 30 min (Note 22). This solution is then cooled to −78 °C and transferred over 2 h via cannula into a 250-mL two-necked, round-bottomed flask equipped with one septum and one low temperature thermometer containing a solution of imide 3 (6.00 g, 18.9 mmol) in THF (80 mL) while maintaining the internal temperature at −78 °C. The resulting mixture is then stirred for 1 h at −78 °C. A solution of methyl cyanoformate (3.00 mL, 37.8 mmol, 2.0 equiv) (Note 23) in THF (10 mL) is then added dropwise via cannula over 15 min. The resulting yellow solution is stirred for 1 h at −78 °C, then the cooling bath is removed, and saturated aqueous NaHCO3 (80.0 mL) is added slowly, followed by de-ionized water (20 mL). The phases are separated, and the aqueous phase is extracted with ethyl acetate (3 × 50 mL). The combined organic phases are washed with brine (50 mL), then dried over MgSO4 (20.0 g, 5 min). After removal of the solid by filtration, the filtrate is concentrated by rotary evaporation. The crude solid residue is dissolved in the minimum amount of CH2Cl2 (approximately 15 mL), charged on a column (8 × 16 cm) containing 400 g of silica gel (Note 12) and eluted with ethyl acetate-petroleum ether (30:70, 1 L) to afford recovered diamine 2 (8.40 g, 96%) (Note 24), and then with AcOEt-petroleum ether (50:50, 2 L) to afford title compound 5 (6.20 g, 87 %) (Note 25).
2. Notes
1.
The checkers used HPLC grade
CH2Cl2 purchased from Fisher and passed through two columns of neutral alumina. The submitters used CH
2Cl
2 freshly distilled over CaH
2 under an Ar atmosphere.
2.
Glyoxal (40% aqueous solution, ~ 8.8 M) was purchased from Fluka Chemical Company.
3.
MgSO4 was purchased from Fisher Company and dried in an oven at 120 °C for 24 h.
4.
(R)-(+)-Phenylethylamine was purchased from Lancaster Chemical Company {99%, 99% e.e. (HPLC)} and used as received.
5.
Throughout this procedure, rotary evaporation refers to a vacuum of 20 mmHg, and high vacuum refers to a vacuum of 1 mmHg.
6.
Crude bis-imine (
1) (R
f = 0.5; petroleum ether:Et
2O 1:1, visualization with KMnO
4)
7.
The apparatus was dried in an oven (120 °C) overnight and maintained under an atmosphere of dry N
2 during the course of the reaction.
8.
The checkers used ACS grade
Et2O stabilized with BHT purchased from Fisher and passed through two columns of neutral alumina. The submitters used anhydrous
Et2O purchased from Fisher Chemicals (water < 0.03%) and purified by pressure filtration under N
2 through activated alumina.
9.
Phenylmagnesium chloride was obtained from Aldrich Chemical Company, Inc.
10.
The submitters performed this addition via cannula.
11.
A white precipitate appeared over the course of the addition.
12.
The checkers used
silica gel 60A (32- 63D) purchased from Bodman Industries. The submitters used
silica gel 60A (35–70μ) purchased from Fluorochem.
13.
The
1H NMR of the crude reaction material indicated a ratio of the title compound (1
S, 2
S)-
2 to the minor isomer (1
R, 2
R)-
2 of 8:2 (R
f (major) = 0.4, (minor) = 0.2, petroleum ether:Et
2O 4:1, visualization with KMnO
4). The minor isomer was identified by a characteristic signal at 3.88 ppm in the
1H NMR,
2 and was isolated containing traces of another isomer (3.83 g, brown oil).
14.
Alternatively, the solution was simply cooled to room temperature, and then left at 4 °C overnight allowing the crystallisation to occur. After collection of a first batch of crystals, the mother liquor was evaporated to 1/5 of its volume to provide a second batch of crystals.
15.
Large colorless crystals. mp = 113–115 °C; [α]
D25 +190.0 (
c = 1.1, CHCl
3); FTIR (CHCl
3): 3322, 2923, 2859, 1602, 1492, 1454, 1363, 1106, 921, 862 cm
−1;
1H NMR
pdf (500 MHz, CDCl
3) δ: 1.25 (d,
J = 6.6 Hz, 6 H), 2.25 (br s, 2 H), 3.37 (s, 2 H), 3.43 (q,
J = 6.4 Hz, 2 H), 6.91–7.24 (m, 20 H);
13C NMR
pdf (125 MHz, CDCl
3) δ: 25.5, 55.1, 65.9, 126.7 (
2), 126.8, 128.0, 128.1, 128.5, 141.8, 145.7; HRMS (ES+)
m/
z calcd for C
30H
33N
2 421.2638, found 421.2644; Found: C, 85.46; H, 7.77; N, 6.64. C
30H
32N
2 requires C, 85.67; H, 7.67; N, 6.66%;
16.
Cyclohexanedicarboxylic anhydride (95%) was purchased from Aldrich Chemical Company, Inc.
17.
2-(3,4-Dimethoxyphenyl)ethylamine (98%) was purchased from Alfa Aesar Company.
18.
The checkers obtained an 82 % yield when the reaction was run on half-scale.
19.
Small white crystals; R
f = 0.4 (petroleum ether:CH
2Cl
2:AcOEt 2:2:1, visualisation with KMnO
4); mp = 86.7–88.1 °C; FTIR (CHCl
3) 3004, 2922, 2860, 1693, 1514, 1399, 1233, 1150, 1025, 807 cm
−1.
1H NMR
pdf (400 MHz, CDCl
3) δ: 1.28 (m, 2 H), 1.37 (m, 2 H), 1.55 (m, 2 H), 1.74 (m, 2 H), 2.72 (m, 2 H), 2.84 (app. t,
J = 7.7 Hz, 2 H), 3.70 (app. t,
J = 7.4 Hz, 2 H), 3.81 (s, 3 H), 3.84 (s, 3 H), 6.71–6.76 (m, 3 H);
13C NMR
pdf (100 MHz, CDCl
3) δ: 21.6, 23.6, 32.9, 39.2, 39.6, 55.8, 55.9, 111.2, 112.0, 121.0, 130.3, 147.7, 148.8, 179.7; MS (EI)
m/
z 317 (M
+, 28%), 164 (C
10H
12O
2, 28%), 151 (C
9H
11O
2, 28%). HRMS: found 317.1634, calcd for C
18H
23NO
4 317.1627. Found: C, 68.22; H, 7.56; N, 4.40. C
18H
23NO
4 requires C, 68.10; H, 7.31; N, 4.42%.
20.
The checkers used HPLC grade
THF purchased from Fisher Chemicals and passed though two columns of neutral alumina. The submitters used anhydrous
THF purchased from Fisher Chemicals (water < 0.03%) and purified by pressure filtration under N
2 through activated alumina.
21.
n-BuLi (2.5 M in hexanes) was purchased from Aldrich Chemical Company, Inc.
22.
The initial color of this solution varied from orange to pink, and after being stirred at room temperature, from pink to purple.
23.
Methyl cyanoformate (99%) purchased from Aldrich Chemical Company, Inc.
24.
The submitters isolated
2 as small white crystals, [α]
D25 +204 (
c = 1.05, CHCl
3).
25.
The checkers obtained an 81% yield when the reaction was run on half-scale. [α]
D25 −52.9 (
c = 1.1, CHCl
3). The submitters obtained an optical rotation of [α]
D25 −62.0 (
c = 1.1, CHCl
3). R
f = 0.4 (petroleum ether:CH
2Cl
2:AcOEt 2:2:1, visualization with KMnO
4); FTIR (CHCl
3) 2940, 1742, 1701, 1515, 1349, 1234, 1026, 805 cm
−1;
1H NMR
pdf (400 MHz, CDCl
3) δ: 1.10 (m, 1 H), 1.30–1.37 (m, 3 H), 1.48–1.60 (m, 2 H), 1.98 (ddd,
J = 14.2, 8.5, 4.2 Hz, 1 H), 2.26 (m, 1 H), 2.86 (app. td,
J = 7.7, 2.2 Hz, 2 H), 3.19 (dd,
J = 6.5, 3.7 Hz, 1 H), 3.72 (s, 3 H), 3.73 (m, 2 H), 3.80 (s, 3 H), 3.83 (s, 3 H), 6.69–6.75 (m, 3 H);
13C NMR
pdf (125 MHz, CDCl
3) δ: 20.5, 20.8, 21.4, 28.5, 32.7, 39.8, 43.8, 53.3, 54.1, 55.9, 56.0, 111.1, 112.0, 121.1, 129.9, 147.8, 148.9, 170.2, 176.1, 177.6; MS (FAB)
m/
z 375 (M
+, 100%), 164 (C
10H
12O
2, 90%), 151 (C
9H
11O
2, 35%); HRMS: found 375.1683, calcd for C
20H
25NO
6 375.1682. Found: C, 63.69; H, 6.75; N, 3.65. C
20H
25NO
6 requires C, 63.99; H, 6.71; N, 3.73. The ee was determined as 86–88% by HPLC (OD column, EtOH:hexanes 10:90, 0.6 mL/min), the retention times were 22.5 min (major) and 27 min (minor). The submitters determined the ee was 93–95% by HPLC (OD column, EtOH:hexanes 10:90, 0.6 mL/min), the retention times were 35 min (major) and 47 min (minor).
Handling and Disposal of Hazardous Chemicals
The procedures in this article are intended for use only by persons with prior training in experimental organic chemistry. All hazardous materials should be handled using the standard procedures for work with chemicals described in references such as "Prudent Practices in the Laboratory" (The National Academies Press, Washington, D.C., 2011 www.nap.edu). All chemical waste should be disposed of in accordance with local regulations. For general guidelines for the management of chemical waste, see Chapter 8 of Prudent Practices.
These procedures must be conducted at one's own risk. Organic Syntheses, Inc., its Editors, and its Board of Directors do not warrant or guarantee the safety of individuals using these procedures and hereby disclaim any liability for any injuries or damages claimed to have resulted from or related in any way to the procedures herein.
3. Discussion
Over the past two decades, the use of chiral lithium amide bases in synthesis has become widespread, enabling the synthesis of chiral building blocks with high selectivity.
3 The three main classes of reactions where chiral lithium amide bases have been applied successfully are: (i) deprotonation of prochiral cyclic ketones, (ii) rearrangement of epoxides to allylic alcohols, and (iii) aromatic and benzylic functionalization of tricarbonyl (η
6-arene)chromium complexes. In all these examples, the chiral base selects between enantiotopic protons in kinetically controlled deprotonations of achiral or prochiral substrates. Another fundamentally different process also exists, in which the chiral lithium amide base first acts as a strong base to produce a prochiral carbanion, e.g. an enolate. The stereochemical outcome of the subsequent reaction of the anion with an electrophile is then controlled by the complexed chiral secondary amine. More recently, catalytic variants of some of the reactions described above have emerged. In this case, a substoichiometric amount of the chiral base is used in conjunction with a stoichiometric amount of an achiral base.
4
The procedure described herein illustrates the efficient use of a chiral lithium amide base to mediate desymmetrization of a prochiral cyclic imide. Imide
5 has been used successfully in a synthesis of the proposed structure of the alkaloid Jamtine.
5 Apart from chiral diamine
2, commercially available bisphenylethylamine in the form of its lithium amide
6 has been used for the desymmetrization of certain imides.
6 However, in many cases chiral base
2 has been shown to provide better selectivity. For example, in the case of imide
9 (Table 1, entry 2), the use of lithium amide
6 proved less selective, providing
9 in only 70% ee. It is also noteworthy that
2 can be used as its mono-lithiated form
4, or as its bis-lithiated derivative
7, both species leading to high enantioselectivities. Table 1 gives examples of other types of imides that have been successfully desymmetrized using chiral base
7.
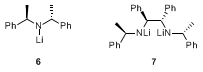
Chiral diamine
2 has also been found to be useful for the desymmetrization of prochiral ketones,
7 piperidines,
8 various tricarbonyl(η
6-arene)chromium complexes,
9 asymmetric rearrangement of episulfoxides,
10 asymmetric thia-Sommelet dearomatization,
11 and asymmetric hydrosilylation
12 with good to excellent levels of enantioselectivities.
The synthesis of diamine
2 suffers from a somewhat low yield; however, it can be easily prepared in large scale.
13 Moreover, it can easily be recovered in essentially pure form by column chromatography after the reaction. Two alternative syntheses of
2 have been reported, either using phenyl lithium instead of a Grignard derivative
2 or
via pinacol coupling of imines.
14 Neither of these methods, however, provided better yields of the product.
In summary, the chiral base desymmetrization of imides provides an efficient method to access highly functionalised molecules with high enantioselectivities. This strategy has been applied to the synthesis of natural products,
5,15,17 as well as a drug molecule.
17
Table 1: Examples of Imide Desymmetrization using Base 7.
Appendix
Chemical Abstracts Nomenclature (Collective Index Number);
(Registry Number)
(R)-(+)-Phenylethylamine:
Benzenemethanamine, α-methyl-, (αR)-; (3886-69-9)
Aqueous glyoxal solution:
Ethandial; (107-22-2)
Phenylmagnesium chloride:
Magnesium, chlorophenyl-; (100-59-4)
1(S),2(S)-Diphenyl-N,N'-bis-[1(R)-phenyl-ethyl]-ethane-1,2-diamine; (156730-49-3)
Cyclohexanedicarboxylic anhydride:
1,3-Isobenzofurandione, hexahydro-; (85-42-7)
2-(3,4-Dimethoxyphenyl)ethylamine:
Benzeneethanamine, 3,4-dimethoxy-; (120-20-7)
(3aS,7aR)-2-[2-(3,4-Dimethoxyphenyl)-ethyl]-hexahydro-isoindole-1,3-dione :
1H-Isoindole-1,3(2H)-dione, 2-[2-(3,4-dimethoxyphenyl)ethyl]-hexahydro-, (3aR,7aS)-rel-; (501085-17-2)
Butyllithium; (109-721-8)
Methyl cyanoformate:
Carbonocyanidic acid, methyl ester (9CI); (17640-15-2)
(3aS,7aS)-2-[2-(3,4-Dimethoxyphenyl)-ethyl]-1,3-dioxo-octahydro-isoindole-3a-carboxylic acid methyl ester; (501085-21-8)
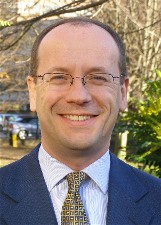 |
Nigel Simpkins was born in 1959 in Luton, England. He completed both his undergraduate studies and his Ph.D (with S. V. Ley) at Imperial College, London. He spent one year with K. C. Nicolaou (Philadelphia) before taking his first lectureship position at Queen Mary College, University of London. In 1988 he moved to the University of Nottingham where he was promoted to professor in 1995. From 2007 he will be Haworth Professor of Chemistry at the University of Birmingham. His research interests include the use of chiral base reagents in synthesis, and the total synthesis of bioactive natural products.
|
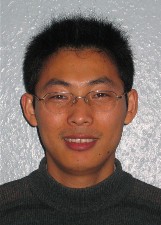 |
Fengzhi Zhang received his B.S. degree in 2000 and M.S. degree in 2003 from Lanzhou University, China where he worked on marine natural product synthesis with Professor Yulin Li. He then worked as a medicinal chemist in a pharmaceutical company in Shanghai until entering the Ph.D. program with an Overseas Research Scholarship at the University of Nottingham in 2004. Under the guidance of Professor Nigel Simpkins his research focuses on the asymmetric synthesis of erythrinan alkaloids.
|
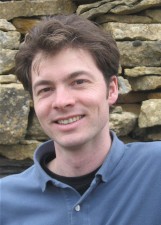 |
Vincent Rodeschini graduated from the School of Chemistry of Mulhouse in 2001. In 2004, he received his PhD from the Université de Haute Alsace, working under the supervision of Prof. Jacques Eustache on the synthesis of angiogenesis inhibitors. He then moved to the UK as a Marie Curie Postdoctoral Fellow at the university of Nottingham, under the mentorship of Prof. Nigel Simpkins (2005–2007). His research was focused on the use of bridgehead lithiation to construct bridgehead substituted natural products. In 2007, he joined the pharmaceutical company Novexel, Paris, as a medicinal chemist in the field of antibiotics.
|
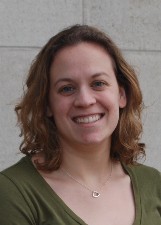 |
Melissa A. Beenen grew up in Oregon and received her BA in chemistry at Northwestern University in 2004. She then began her doctoral studies at the University of California, Berkeley in the laboratories of Professor Jonathan A. Ellman. Her graduate research has focused on the metal-catalyzed asymmetric synthesis of amines using N-tert-butanesulfinamde.
|
Copyright © 1921-, Organic Syntheses, Inc. All Rights Reserved