Org. Synth. 2010, 87, 16
DOI: 10.15227/orgsyn.087.0016
REGIOSELECTIVE C-4 BROMINATION OF OXAZOLES: 4-BROMO-5-(THIOPHEN-2-YL)OXAZOLE
Submitted by Bryan Li
1, Richard A. Buzon, and Zhijun Zhang.
Checked by Takahiro Koshiba and Tohru Fukuyama.
1. Procedure
A. 5-(Thiophen-2-yl)oxazole (
1).
2 A 1-L three-necked, round-bottomed flask equipped with a reflux condenser fitted with an argon inlet, a thermocouple probe (range -200 to +1370 °C) and a mechanical stirrer is charged with
2-thiophenecarboxaldehyde (11.21 g, 100.0 mmol, 1.00 equiv),
K2CO3 (24.19 g, 175.0 mmol, 41.75 equiv),
tosylmethylisocyanide (21.12 g, 100.0 mol, 1.00 equiv) and
methanol (448 mL) (Note 1). The reaction mixture is heated at reflux (66 °C) for 4 h
(Note 2). After cooling to room temperature,
water (224 mL) is added and the mixture is stirred for 10 min at the same temperature. The resulting solution is transferred to a single-necked 2-L flask, and methanol is removed by rotary evaporation (45 °C, 75 mmHg). The residual liquid is transferred to a 1-L separatory funnel, and then extracted with
MTBE (3 × 120 mL). The combined organic layers are washed with
water (1 × 50 mL) and a saturated
NaCl solution (1 × 50 mL), dried over anhydrous MgSO
4, filtered, and concentrated by rotary evaporation (35 °C, 50 mmHg)
(Note 3) to give a brown oil (15.31 g) as a crude product, which is vacuum distilled (60-62 °C, 0.4 mmHg) to afford the oxaozle
1 as a pale yellow oil (12.70 g, 84%)
(Note 4).
B. 4-Bromo-5-(thiophen-2-yl)oxazole (2). A dried 300-mL, three-necked, round-bottomed flask equipped with a 75-mL pressure equalizing addition funnel fitted with an argon inlet, a temperature probe and a mechanical stirrer (stirring rate >250 rpm) is charged with 5-(thiophen-2-yl)oxazole (1) (9.14 g, 60.5 mmol, 1.00 equiv) and anhydrous DMF (36.6 mL) (Note 5). The resulting solution is cooled to a -15 °C to -10 °C range (Note 6), and 1 N lithium bis(trimethylsilyl)amide in THF (1.0 M solution, 63.5 mL, 1.05 equiv) (Note 7) is then added slowly via a syringe while keeping the reaction temperature at -15 °C to -10 °C. The reaction mixture is stirred at -15 °C for 30 min and then cooled to -78 °C (dry ice-acetone bath). A solution of N-bromosuccinimide (NBS) (10.76 g, 60.45 mmol, 1.00 equiv) in anhydrous DMF (36.6 mL) (Note 8) is added while maintaining the temperature below -65 °C (Note 9). The mixture is stirred for 30 min at the same temperature, and quenched by addition of 2 N aqueous NaOH solution (160 mL) while allowing the solution to warm to above 0 °C during the quench (Note 10). After diluting with water (160 mL), the reaction is extracted with MTBE (3 × 120 mL). The combined organic layers are washed successively with a 0.5 N NaOH solution (3 × 50 mL) and a saturated NaCl solution (1 × 50 mL), dried over anhydrous MgSO4, filtered, and concentrated by rotary evaporation (35 °C, 115 mmHg) (Note 11) to afford a brown solid as the crude product. The solid is then dissolved in isopropyl alcohol (30 mL) with gentle warming (60 °C water bath). The resulting solution is cooled to room temperature over 20 min and further cooled in an ice-bath (without agitation). After stirring for 30 min with ice bath cooling, the resulting crystals are collected by suction filtration on a Kiriyama funnel, rinsed with ice-cold isopropyl alcohol (5 mL), and transferred to a 250-mL Erlenmeyer flask. The filtrate is transferred to a 50-mL, single-necked, round-bottomed flask, and concentrated to ~10 mL by rotary evaporation (40 °C, 50 mmHg). The concentrated filtrate is cooled to room temperature over 20 min and further cooled in a (water)ice-acetone bath for 30 min (without agitation). The resulting crystals are collected by suction filtration on a Kiriyama funnel, rinsed with (water)ice-acetone cold isopropanol (3 mL), and transferred to the same 250-mL Erlenmeyer flask. Heptanes (40 mL) are charged to the Erlenmeyer flask, the resulting mixture is stirred for 10 min, and filtered with a Kiriyama funnel. The collected product is dried in a desiccator (room temperature/ < 1.0 mmHg) (Note 12) for 18 h to give 4-bromo-5-(thiophen-2-yl)oxazole (2) (11.01 g, 79% ) (Note 13) as an off-white solid.
2. Notes
1.
Unless otherwise noted, all reagents were purchased from Aldrich Chemical Company and used without further purification. The checkers purchased anhydrous methanol and TosMIC from Wako chemicals and Tokyo chemical industry company, respectively, and used them as received.
2.
The reaction turned brown after 4 h at reflux. According to the submitters, HPLC analysis of a reaction aliquot showed complete consumption of 2-thiophenecarbaldehyde. HPLC method: Agilent
® Eclipse XDB-C8 columns (4.6 mm × 150 mm). Mobile phase: acetonitrile (A) / 0.2% perchloric acid in water (B), 0-2 min, isocratic 70% B; 2-10 min, gradient from 70% B to 10% B. Flow rate: 2 mL/min. Detection wavelength: 210 nm. The HPLC retention times for 5-(thiophen-2-yl)oxazole (
1) and 4-bromo-5-(thiophen-2-yl)oxazole (
2) were 6.37 min and 7.84 min, respectively. The checkers could detect the consumption of the starting material by TLC.
3.
The submitters reported the pressure at 20 mmHg.
4.
The physical properties of 5-(thiophen-2-yl)oxazole (
1) are as follows: bp 60-62 °C (0.40 mmHg) [submitter: bp 66-68 °C (0.60 mmHg)]; IR (neat): 3111, 1614, 1593, 1517, 1486, 1425, 1364, 1305, 1258, 1241, 1210, 1197, 1101, 991, 899, 848, 818, 700, 637 cm
-1;
1H NMR
pdf (400 MHz, CDCl
3) δ: 7.09 (dd,
J = 4.8, 3.6 Hz, 1 H), 7.22 (s, 1 H), 7.33 (dd,
J = 3.6, 1.1 Hz, 1 H), 7.35 (dd,
J = 4.8, 1.1 Hz, 1 H), 7.86 (s, 1 H);
13C NMR
pdf (100 MHz, CDCl
3) δ: 121.0, 124.4, 125.7, 127.7, 129.3, 146.8, 149.7; HRMS (DART)
m/z calcd. for C
7H
6NOS [M+H]
+ 152.0170; found 152.0165; Anal. calcd. for C
7H
5NOS: C, 55.61; H, 3.33; N, 9.26; found: C, 55.44; H, 3.57; N, 9.22. The submitters determined the purity of
2 to be 98.8% by HPLC analysis (210 nm). The HPLC method is the same as in
Note 2.
5.
Anhydrous DMF was purchased from Wako Chemical.
6.
Dry ice-acetone bath with limited amount of dry ice to keep the temperature in range.
7.
Lithium bis(trimethylsilyl)amide (1.0 M in THF) supplied in SureSeal bottles was purchased from Aldrich Chemical Company. The submitters recommend that charging the reagent by weight is preferred over charging by volume for higher accuracy.
8.
To minimize the transfer of the NBS in DMF solution, solid NBS is directly added to the 100-mL pressure equalizing addition funnel with the bottom of the funnel attached to a 10-mL single-necked round bottom flask and the top capped with a septum fitted with an argon inlet, DMF is charged to the funnel via syringe. The mixture is swirled into a complete solution, the 10-mL single-necked round bottom flask is removed, and the addition funnel is attached to the 300-mL, three-necked reaction flask.
9.
Although the freezing point of DMF is -61 °C, the reaction mixture does not freeze at -78 °C. Best regioselectivity is obtained when the NBS in DMF solution is allowed to trickle down the sidewall of the reaction flask with good agitation of the reaction mixture (stirring rate >250 rpm). The reaction is exothermic, and addition is completed in approx. 30 min.
10.
The quench was complete in 3 min. The dry ice-acetone bath was removed immediately after the quench.
11.
The submitters reported that the resulting solution was concentrated by rotary evaporation (35 °C, 20 mmHg).
12.
The submitters used a vacuum oven (40 to 50 °C/ ca. 75 mmHg) to dry the collected product.
13.
The physical properties of 4-bromo-5-(thiophen-2-yl)oxazole (
2) are as follows: mp 85-87 °C; IR (neat): 3120, 1603, 1501, 1486, 1427, 1319, 1169, 1116, 1062, 1027, 932, 915, 850, 831, 706, 642 cm
-1;
1H NMR
pdf (400 MHz, CDCl
3) δ: 7.14 (dd,
J = 5.0, 3.7 Hz, 1 H), 7.43 (dd,
J = 5.0, 1.4, 1 H), 7.61 (dd,
J = 3.7, 1.4 Hz, 1 H), 7.80 (s, 1 H);
13C NMR
pdf (100 MHz, CDCl
3) δ: 110.0, 125.9, 126.8, 127.7, 127.8, 144.0, 149.0; HRMS (ESI)
m/z calcd. for C
7H
5BrNOS [M+H]
+ 229.9275; found 229.9271; Anal. calcd. for C
7H
4BrNOS: C, 36.54; H, 1.75; N, 6.09; found: C, 36.61; H, 1.91; N, 6.10. The submitters determined the purity of
2 to be 98.9% by HPLC analysis (210 nm). The HPLC method is the same as in
Note 2.
Handling and Disposal of Hazardous Chemicals
The procedures in this article are intended for use only by persons with prior training in experimental organic chemistry. All hazardous materials should be handled using the standard procedures for work with chemicals described in references such as "Prudent Practices in the Laboratory" (The National Academies Press, Washington, D.C., 2011 www.nap.edu). All chemical waste should be disposed of in accordance with local regulations. For general guidelines for the management of chemical waste, see Chapter 8 of Prudent Practices.
These procedures must be conducted at one's own risk. Organic Syntheses, Inc., its Editors, and its Board of Directors do not warrant or guarantee the safety of individuals using these procedures and hereby disclaim any liability for any injuries or damages claimed to have resulted from or related in any way to the procedures herein.
3. Discussion
Oxazoles with substitutions at both C-4 and C-5 have recently attracted increased attention in medicinal chemistry research for their therapeutic potential in treating inflammation, cancer, and asthma.
3 We recently engaged in the development of a scaleable preparation of
3, a potent and selective inhibitor of the stress-activated kinase p38α.
4 Our strategy was to employ a Suzuki-Miyaura or Negishi coupling as shown in
Scheme 1. In this approach, a robust and efficient synthesis of 4-halo-5-substituted oxazoles is a critical component.
Scheme 1
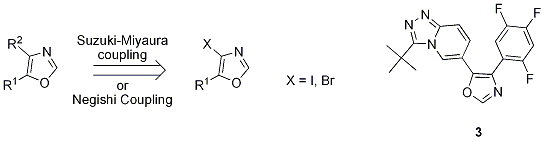
C-4 iodination of 5-substituted oxazoles was first reported by Vedejs
et al.
5 However, the regioselectivity (C-4/C-2) of the reaction was modest, both 2-iodooxazoles and 2,4-diiodooxazoles were formed as by-products at significant levels. It is known in the literature that the regioselectivity of this reaction is determined by the equilibrium between the 2-lithiooxazole and its acyclic tautomer
(
Scheme 2).
6,7 In polar aprotic solvents, the acyclic form is expected to predominate because of improved solvation. We envisioned that DMF, as a strongly polar aprotic solvent, would be an ideal solvent in this reaction. It is cheap, commercially available in anhydrous form and most importantly, it has a low freezing point of -61 °C. Other common polar aprotic solvents were ruled out because of their high freezing points (DMAC, NMP, DMSO, DMI, acetonitrile), ability to react with anions (acetone, acetonitrile, DMSO), cost (DMI, DMPU), and worker safety and environmental concerns (HMPT, HMPA).
8
Scheme 2
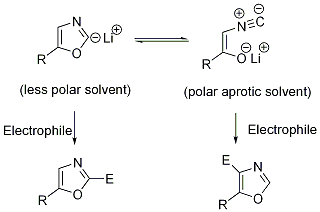
Our initial attempts employing DMF as solvent at -70 °C gave poor to modest C-4/C-2 regioselectivity. We reasoned that the initially formed 2-lithiooxazole did not have ample opportunity to fully equilibrate to the acyclic form at low temperatures within the time scale of the reaction. We therefore decided to perform the lithiation at -15 °C, equilibrate the anion for 30 min before cooling to -70 °C and quenching with NBS. Employing these conditions, a regioselectivity of 98:2 favoring the desired C-4 bromide was obtained. The product (Table 1, entry 1) was isolated by crystallization (MTBE/hexanes) in 87% yield and contained less than 0.2% of 2-bromooxazole as an impurity. As expected, the lithium anions behave similarly to stabilized enolates in that we did not detect any formylated products (from nucleophilic addition to DMF) in the reaction mixtures. The regioselective C-4 bromination is general (Table 1); excellent regioselectivity (> 97:3) was observed using other C-5 substituted aryl and heteroaryl oxazoles as substrates under the same reaction conditions.
Table 1. C-4 Bromination of 5-Substituted Oxazoles via 2-Lithiooxazoles.
Table 2. Suzuki-Miyaura Coupling Reactions of 4-Bromooxazoles.
4-Bromooxazoles are known to be good substrates for Suzuki-Miyaura coupling reactions. Coupling with aryl boronic acids in the presence of Pd(dppf)Cl2 proceeded uneventfully (Table 2).
In conclusion, a highly regioselective bromination at C-4 of 5-substituted oxazoles is described. The use of DMF as solvent and aging of the lithiated oxazole are critical to drive the equilibrium in favor of the acyclic isonitrile enolate, resulting in significantly improved C-4/C-2 regioselectivity. These 4-bromooxazoles were shown to be good Suzuki-Miyaura coupling partners with arylboronic acids. The bromination protocol was demonstrated on multi-kilogram scale supporting the development of 3.
Appendix
Chemical Abstracts Nomenclature (Collective Index Number);
(Registry Number)
5-(Thiophen-2-yl)oxazole: Oxazole, 5-(2-thienyl)-; (70380-70-0)
2-Thiophenecarboxaldehyde; (98-03-3)
Tosylmethylisocyanide: Benzene, [(isocyanomethyl)sulfonyl]-; (36635-63-9)
4-Bromo-5-(thiophen-2-yl)oxazole: Oxazole, 4-bromo-5-(2-thienyl)-; (959977-82-3)
Lithium bis(trimethylsilyl)amide; (4039-32-1)
N-Bromosuccinimide: 2,5-Pyrrolidinedione, 1-bromo-; (
128-08-5)
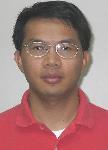 |
Bryan Li was born in 1966. He received a B.S. from the Department of Fine Chemical Technology, East China University of Chemical Technology in 1986. After three years of working in Amoy Pharmaceutical Inc. at Xiamen, China. He pursued his graduate education in chemistry at the University of Rhode Island under the guidance of Prof. Elie Abushanab, and received a Ph.D. in 1993. In the following two years, he worked in Prof. Gary Posner's group at the Johns Hopkins University at Baltimore, Maryland as a postdoctoral fellow. In 1996, he moved overseas and worked in the National University of Singapore, and returned to the U.S. in 1998. He has since worked in Pfizer Inc (Groton, CT) as a process chemist, and serves as a program lead responsible for exploratory development of drug candidates.
|
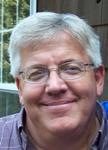 |
Rich Buzon started at Pfizer in 1979 in the Specialty Chemical Group as a chemical operator. After seven years in various positions in Specialty Chemicals, he began taking Organic Chemistry classes at the University of Connecticut and also at Connecticut College. He transferred to the Process Research and Development department in 1992 and began enabling process development for project chemistry to 22L glassware and 500 gal Pilot Plant reactor size. He is currently in the Research API group at Pfizer Global Research and Development.
|
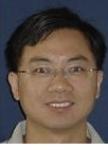 |
Zhijun Zhang (born 1971) received his B.S. from Nanjing University, China in 1993 and M.A. from Bryn Mawr College, Pennsylvania in 2000. He has since worked with Pfizer Inc. in a few roles. He started as a medicinal chemist with Pharmacia Corp, Kalamazoo, Michigan. After the acquisition, he transferred to Pfizer Groton and later joined the Research API group and worked on process chemistry. He is now in the Research Analytical group, working on API/drug release and PAT (Process Analytical Technology) projects.
|
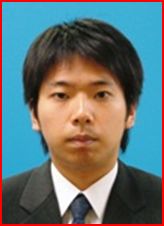 |
Takahiro Koshiba was born in 1981 in Saitama, Japan. He received B.S. in 2004 from university of the Meiji Pharmaceutical University and M.S. in 2006 from the University of Tokyo. Presently, he is pursuing Ph.D. degree at the Graduate School of Pharmaceutical Sciences, the University of Tokyo, under the guidance of Professor Tohru Fukuyama. His research interests are in the area of the total synthesis of natural product.
|
Copyright © 1921-, Organic Syntheses, Inc. All Rights Reserved