Org. Synth. 2011, 88, 353-363
DOI: 10.15227/orgsyn.088.0353
TANDEM NUCLEOPHILIC ADDITION / FRAGMENTATION OF VINYLOGOUS ACYL TRIFLATES: 2-METHYL-2-(1-OXO-5-HEPTYNYL)-1,3-DITHIANE
Submitted by Marilda P. Lisboa, Tung T. Hoang, and Gregory B. Dudley
1.
Checked by Jimmie Weaver and Jonathan Ellman.
1. Procedure
Caution: Exercise care in the handling of trifluoromethanesulfonic anhydride (corrosive), pyridine (harmful by inhalation), and butyllithium (flammable, corrosive).
A. 2-Methyl-3-trifluoromethansulfonyloxy-cyclohex-2-en-1-one. An oven-dried 2-L, three-necked, round-bottomed flask equipped with a 1 inch oval magnetic stir bar, a rubber septum, nitrogen inlet and thermometer is charged with 2-methyl-1,3-cyclohexanedione (15.0 g, 0.115 mol, 1.0 equiv) (Note 1), pyridine (19.0 mL, 0.230 mol, 2 equiv) (Note 2) and methylene chloride (700 mL) (Note 3).
The reaction mixture is magnetically stirred, cooled at -78 °C for 20 min (Note 4), and trifluoromethanesulfonic anhydride (23.5 mL, 0.138 mol, 1.2 equiv) (Note 1) is added dropwise via syringe over 10 min (Notes 5 and 6).
The light yellow reaction mixture is stirred at -78 °C for 20 min, at 0 °C for 20 min, and at room temperature for 30 min (Note 4), at which time the reaction mixture is a homogeneous red solution.
The reaction mixture is acidified using 200 mL of a 1M aqueous solution of hydrochloric acid (Note 7), and the resulting biphasic solution is transferred to a 2-L separatory funnel.
The organic phase is removed, and the aqueous layer is extracted with diethyl ether (3 x 200 mL) (Note 8).
The organic phases are combined and dried over 100 g of Na2SO4 and filtered by vacuum suction through a medium porosity fritted glass funnel, and the solvent is removed under reduced pressure by rotary evaporation (25-45 mmHg, room temperature water bath) providing the crude product as a red oil (Note 9).
The crude product is purified by chromatography on silica gel (Note 10) to afford 30.0 g (98%) of the product as a colorless oil (Note 11).
2-Methyl-3-trifluoromethansulfonyloxy-cyclohex-2-en-1-one is indefinitely stable to storage under nitrogen at -13 °C.
B. 2-Methyl-2-(1-oxo-5-heptynyl)-1,3-dithiane. An oven-dried two-necked, 500-mL, round-bottomed flask equipped with 0.5 inch oval magnetic stir bar, rubber septum, and a nitrogen inlet adapter is charged with 2-methyl-1,3-dithiane (Note 12) (4.64 mL, 38.7 mmol, 1.1 equiv) and tetrahydrofuran (150 mL) (Note 13).
The resulting solution is cooled at -78 °C (Note 4).
A solution of n-butyllithium (Note 14) (14.5 mL, 35.2 mmol, 1.0 equiv) is added dropwise via syringe over 10 min (Note 15).
The light yellow reaction mixture is stirred at -78 °C for 20 min, at 0 °C for 10 min, and at -30 °C for 15 min (Note 4), and then 2-methyl-3-trifluoromethanesulfonyloxy-cyclohex-2-en-1-one (10.0 g, 38.7 mmol, 1.1 equiv) is added dropwise by syringe over 10 min.
The light yellow reaction mixture is stirred at -30 °C for 15 min, at 0 °C for 15 min, and at room temperature for 30 min (Note 4), at which time the reaction mixture is a homogeneous red solution (Note 16).
A half-saturated solution of aqueous ammonium chloride (100 mL) (Note 17) is then added and the biphasic solution is transferred to a 500-mL separatory funnel and extracted with diethyl ether (3 x 50 mL) (Note 8).
The organic phases are combined and dried over 70 g of Na2SO4, filtered by vacuum suction through a medium porosity fritted glass funnel, and the solvent is removed under reduced pressure by rotary evaporation (25-45 mmHg, room temperature water bath) providing the crude product as a yellow oil.
The crude product is purified by chromatography on silica gel (Note 18) to afford 6.64 g (71%) (Note 19) of the product as a colorless oil (Note 20).
2. Notes
1.
2-Methyl-1,3-cyclohexanedione (97%) and
trifluoromethane-sulfonic anhydride (99%) were purchased from Sigma-Aldrich and used without further purification.
2.
Pyridine (99%) was purchased from Sigma-Aldrich and stored over KOH pellets.
3.
Methylene chloride (HPLC Grade) was purchased from Fischer Scientific and purified using an Innovative Technology solvent purification system.
4.
The indicated temperatures at which reaction mixtures are kept refer to the temperature of external cooling baths as follows: (a) -78 °C cooling bath prepared with dry ice and acetone, (b) 0 °C cooling bath prepared with ice and water, and (c) -30 °C cooling bath prepared by adding dry ice to acetone and carefully monitoring the bath temperature.
No external bath was employed for reactions conducted at room temperature.
5.
The highest internal temperature observed by the checkers was
-61 °C.
6.
The submitters observed that a small amount of white precipitate forms during the addition of trifluoromethanesulfonic anhydride.
7.
Hydrochloric acid (1 M) was prepared by dilution of hydrochloric acid (12.1 M, ACS grade) purchased from EMD Chemicals.
8.
Ethyl ether (anhydrous, ACS grade) was purchased from EMD Chemicals and used without further purification.
9.
The submitters obtained a yellow oil.
10.
The crude product was purified using 300 g of Silica Gel 60 (Geduran(r) 40-63 µM) wet-loaded as a slurry.
The sample was loaded in a minimum volume of eluent, passed through the column using gradient
elution (500 mL of 9:1 hexanes:EtOAc, 500 mL of 8:2 hexanes:EtOAc, and 1000 mL of 7:3 hexanes:EtOAc), and collected in 20-mL fractions.
Fractions 58-99 were collected, combined, and concentrated under reduced pressure to obtain the final product.
The R
f of 2-methyl-3-trifluoromethansulfonyloxy-cyclohex-2-en-1-one = 0.62 and 2-methyl-1,3-cyclohexanedione = 0.22 in 8:2 hexanes:ethyl acetate.
11.
The compound exhibited the following physical and spectroscopic data: IR (neat): 2964, 1688, 1416, 1344, 1210, 1135, 1024, 912, 891, 793, 759 cm
-1.
1H NMR
pdf(500 MHz, CDCl
3) δ: 1.77 (d,
J = 1.9 Hz, 3 H), 2.05 - 1.97 (apparent pentet, 2 H), 2.40 (t,
J = 7.9 Hz, 2 H), 2.66 (dtd,
J = 6.1, 4.0, 1.9 Hz, 2 H);
13C NMR
pdf(126 MHz, CDCl
3) δ: 9.0, 20.5, 28.6, 36.5, 118.2 (q,
JCF = 319.8 Hz), 127.9, 162.0, 197.5; HRMS (ESI) calcd.
for C
8H
9HF
3O
4S[M+H]: 259.0246.
Found: 259.0246.
Anal.
calcd.
for C
8H
9F
3O
4S: C, 37.21; H, 3.51; F, 22.07; S, 12.42.
Found: C, 37.32; H, 3.45; F, 21.84; S, 12.41.
12.
2-Methyl-1,3-dithiane (=98%) was purchased from Fluka and used without further purification.
13.
Tetrahydrofuran (HPLC Grade) was purchased from EMD Chemicals and purified using an Innovative Technology solvent purification system.
14.
n-Butyllithium (2.5 M solution in hexanes) was purchased from Sigma-Aldrich and titrated using (-)-menthol and 1,10-phenanthroline (= 99%).
15.
The internal temperature reached -57 °C during addition.
16.
TLC analysis showed formation of product by TLC (R
f (reaction product) = 0.3; R
f (2-methyl-1,3-dithiane) = 0.40, R
f (2-methyl-3-trifluoromethansulfonyloxy-cyclohex-2-en-1-one) = 0.2; elution with 9:1 hexanes:EtOAc; UV and KMnO
4/heating visualization).
Completion of the reaction is difficult to discern because
n-butyllithium is the limiting reagent, and therefore both the dithiane and triflate remain even after all of the butyllithium has been consumed.
17.
Half-saturated aqueous ammonium chloride solution was prepared by dilution of a saturated aqueous ammonium chloride solution;
ammonium chloride (99.5% ACS) reagent purchased from Sigma-Aldrich.
18.
The crude product was purified using 300 g of Silica Gel 60 (Geduran(r) 40-63 µM) wet-loaded as a slurry.
The sample was loaded in a minimum volume of eluent, passed through the column using isocratic elution (2000 mL of 98:2 hexanes:EtOAc), and collected in 20-mL fractions.
Fractions 38-85 were collected, combined, and concentrated under reduced pressure to obtain the final product.
The checkers found that excess dithiane complicated isolation of the product.
However, most of the excess dithiane could be easily removed by placing the unpurified reaction product under high vacuum overnight.
The checkers were then able to isolate the product using 360 g of silica and 3.5 L of 95:5 hexanes:EtOAc.
Fractions of 25 mL were collected, and the product was found in fractions 55-93.
19.
The checkers observed that yields were improved substantially using freshly distilled dithiane (55 °C, 1 mmHg), recently chromatographed triflate that was concentrated from anhydrous THF, and a newly opened bottle of butyllithium in hexanes.
20.
The compound exhibited the following physical and spectroscopic data: IR (neat): 2918, 1700, 1446, 1423, 1372, 1359, 1277, 1243, 1217, 1149, 1071, 998, 907, 868, 673 cm
-1.
1H NMR
pdf(400 MHz, CDCl
3) δ: 1.68 (s, 3 H), 1.87 - 1.71 (m, 6 H), 2.14 - 2.04 (m, 1 H), 2.18 (td,
J = 6.8, 2.6 Hz, 2 H), 2.62 (dt,
J = 7.5, 3.5 Hz, 2 H), 2.79 (t,
J = 7.2 Hz, 2 H), 3.10 (t,
J = 13.7 Hz, 2 H);
13C NMR
pdf(126 MHz, CDCl
3) δ: 3.6, 18.2, 24.1, 24.3, 25.0, 28.2, 34.6, 54.8, 76.5, 78.3, 203.9.
HRMS (ESI) calcd.
for C
12H
18HOS
2[M+H]: 243.0872.
Found: 243.0873.
Anal.
calcd.
for C
12H
18OS
2: C, 59.46; H, 7.48; S, 26.46.
Found: C, 59.48; H, 7.25; S, 26.59.
Safety and Waste Disposal Information
All hazardous materials should be handled and disposed of in accordance with "Prudent Practices in the Laboratory"; National Academy Press; Washington, DC, 1995.
3. Discussion
Alkyne building blocks offer many advantages in chemical synthesis owing to their high enthalpic (chemical potential energy) content and orthogonal reactivity with respect to other common organic functional groups.
2 The advent of late transition metal catalysts that display a preference for coordination to π-systems has expanded the scope of alkyne reactions and further increased their value.
3
As the scope of alkyne reactions continues to expand, so too does the demand for efficient synthetic methods that produce key alkyne building blocks.
However, the energy-rich alkyne π-system is not easy to generate.
Functionalized alkynes are typically prepared using methods that make use of simpler alkyne starting materials, like acetylide coupling.
Common methods for the generation of alkyne π-bonds from non-acetylenic starting materials include elimination, vinylidene carbene rearrangement, and fragmentation reactions.
4
Vinylogous acyl triflates (VATs) undergo tandem nucleophilic addition / fragmentation reactions to provide tethered keto-alkynes.
5 The overall sequence achieves the conversion of symmetric, cyclic diones into acyclic alkynyl ketones that comprise orthogonal and non-contiguous functionalities.
The stability of the triflate anion (an excellent leaving group) enables the fragmentation process in much the same way as formation of molecular nitrogen is a driving force in the classic Eschenmoser-Tanabe reaction.
6 These mechanistically related reactions are among the few C-C bond cleaving fragmentations that produce alkyne π-systems.
7
Scheme 1. Alternative strategies for the synthesis of (Z)-6-heneicosen-11-one
The utility of carbanion-triggered fragmentation of vinylogous acyl triflates as a modern alternative to the Eschenmoser-Tanabe fragmentation is illustrated in the synthesis of (
Z)-6-heneicosen-11-one, the sex pheromone of the Douglas fir tussock moth (Figure 1).
In an earlier approach,
8 a cyclic vinylogous methyl ester was treated with
n-decyl Grignard in an addition-hydrolysis sequence to set up the multi-stage Eschenmoser-Tanabe process.
Using the current method, nucleophilic addition of the same Grignard reagent to the corresponding vinylogous triflate triggered fragmentation directly.
9 The streamlined entry into the fragmentation pathway provides an increased overall yield in fewer steps, and it avoids acidic conditions and the sometimes-troublesome preparation of cyclic enone oxides.
6b
The scope of appropriate nucleophiles is illustrated in Table 1.
Carbanion reagents were the first nucleophiles to be explored,
5 as these provide alkyl and aryl ketones of the type most closely associated with the Eschenmoser-Tanabe fragmentation (entries 1-4, 10-12).
The typical reaction is conducted by treating the nucleophile with the vinylogous acyl triflate in THF at low temperature to achieve the initial 1,2-addition, and then warming the reaction mixture to room temperature or above to promote the (entropically favorable) fragmentation event.
It has been observed experimentally that addition / fragmentation reactions using unstabilized
Table 1. Scope of the addition/fragmentation reaction
alkyllithium and alkyl Grignard reagents occur more efficiently in toluene than in THF (entry 2);
9 this observation may be attributable to solvent-dependent aggregation states of the organometallic reagent.
In toluene, higher aggregation states of the nucleophile likely attenuate its reactivity and promote selectivity for the desired 1,2-addition over alternative pathways like proton- or electron-transfer.
Addition of stabilized carbanion nucleophiles to vinylogous acyl triflates triggers what we term the VAT-Claisen
10 reaction: a ring-opening fragmentation to give acyclic β-keto ester and related products much like those observed traditionally in the Claisen condensation (entries 5-6).
Whereas the Claisen condensation is driven by deprotonation of final product, the VAT-Claisen reaction is rendered irreversible by the fragmentation event.
Deprotonation of the final VAT-Claisen product may still occur incidentally, in which case the use of excess nucleophile is required to realize complete conversion (entry 5).
Other suitable nucleophiles for promoting fragmentation include primary lithium amides (entry 7) and lithium triethylborohydride (entry 8).
5 Diisobutylaluminum hydride reduces the VAT carbonyl but does not trigger fragmentation; the resulting alcohol can then be treated with excess Grignard reagents to promote fragmentation and in situ trapping of the resultant aldehyde (entry 9).
11 Other suitable VAT substrates are shown in entries 10-12.
The five- and seven-membered ring VATs are less stable than their hexacyclic counterparts, and as such they are best prepared immediately prior to use.
The tandem nucleophilic addition / fragmentation of cyclic VAT substrates provides an alternative entry into the classic Eschenmoser-Tanabe pathway, one of the few Grob-type fragmentations capable of generating alkyne π-bonds.
The method outlined above delivers orthogonally functionalized acyclic building blocks - alkynyl ketones and related compounds - from symmetrical cyclic dione starting materials.
This methodology continues to evolve; application to the synthesis of natural products,
12 homopropargyl alcohol
13 and homopropargyl amine
14 derivatives from heterocyclic VATs, and other challenging classes of organic compounds
15 are underway at this time.
Appendix
Chemical Abstracts Nomenclature (Collective Index Number);
(Registry Number)
2-Methyl-2-(1-oxo-5-heptynyl)-1,3-dithiane: 5-Heptyn-1-one, 1-(2-methyl-1,3-dithian-2-yl)-; (892874-94-1)
2-Methyl-1,3-dithiane; (6007-26-7)
n-Butyllithium; (109-72-8)
2-Methyl-3-trifluoromethansulfonyloxy-cyclohex-2-en-1-one: Methanesulfonic acid, 1,1,1-trifluoro-, 2-methyl-3-oxo-1-cyclohexen-1-yl ester; (150765-78-9)
2-Methyl-1,3-cyclohexanedione; (1193-55-1)
Trifluoromethanesulfonic anhydride; (358-23-6)
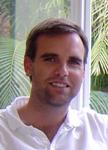 |
Gregory B.
Dudley received a B.A.
from FSU in 1995 and a Ph.D.
in 2000 under the direction of Professor Rick Danheiser at MIT.
After completing an NIH Postdoctoral Fellowship with Professor Samuel Danishefsky at the Sloan-Kettering Institute for Cancer Research, he returned to FSU as an assistant professor in 2002.
Professor Dudley was promoted to associate professor with tenure in 2008.
The current mission of his research program is to impact the drug discovery process by contributing fundamental new knowledge in organic synthesis.
The addition / fragmentation methodology featured herein has figured prominently in this effort over the past few years.
|
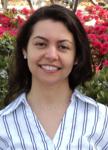 |
Marilda P.
Lisboa was born in Rio de Janeiro and raised in Minas Gerais, Brazil.
She received her B.S.
degree in pharmacy in 2005 and M.S.
degree in pharmaceutical sciences in 2007 from the Federal University of Minas Gerais, Brazil.
In 2008, she was awarded a Capes-Fulbright fellowship to pursue her PhD in Chemistry at Florida State University.
Currently, she is a PhD student under the supervision of Professor Gregory Dudley and her research is focused on the synthesis of the natural product palmerolide A.
|
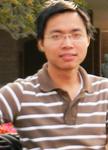 |
Tung T.
Hoang was born in Ha Long, a small yet beautiful city known for the Ha Long Bay with thousands of islets and its sunshine beach in northeast Vietnam.
Inspired by his high school teacher, Tung entered the chemistry program of Vietnam National University where he earned his B.A.
degree in 2006.
As a recipient of the Vietnam Education Foundation Fellowship cohort 2009, he went to Florida State University to pursue his PhD in organic chemistry.
Tung is currently working under the direction of Professor Gregory Dudley with interest in the total syntheses of biologically active natural products.
|
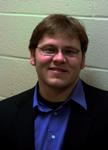 |
Jimmie Weaver was born in 1981 in Oklahoma (US).
He studied at Southern Nazarene University where he obtained his B.S.
degree in chemistry in 2004.
After spending the next year working under William Hildebrand (OUHSC) in an immunology lab, he began his graduate studies at the University of Kansas under the supervision of Jon Tunge.
The primary focus of his graduate work was the synthesis and decarboxylative coupling of a-sulfonyl acetic esters.
He completed his Ph.D.
in the spring of 2010 and began a post-doctoral fellowship under the direction of Jon Ellman (Yale) where he is currently working on organocatalysis and drug delivery projects.
|
Copyright © 1921-, Organic Syntheses, Inc. All Rights Reserved