Org. Synth. 2013, 90, 190-199
DOI: 10.15227/orgsyn.090.0190
Bimolecular Oxidative Amidation of Phenols: 1-(Acetylamino)-4-oxo-2,5-cyclohexadiene-1-acetic acid, Methyl Ester
Submitted by Jaclyn Chau
1 and Marco A. Ciufolini*
1.
Checked by Aaron Bedermann and John L. Wood.
1. Procedure
A. Methyl 2-(1-acetamido-4-oxocyclohexa-2,5-dien-1-yl)acetate (2). An oven-dried (110 °C), three-necked, 1-L round-bottomed flask is equipped with a 1.25-inch egg-shaped magnetic stirring bar and three rubber septa, one containing a temperature probe and another a nitrogen inlet. Solid (diacetoxyiodo)benzene ("DIB," 23.9 g, 74.2 mmol, 1.35 equiv) (Note 1) is added under purging N2 followed by CH3CN (480 mL) (Note 2). The flask is purged for 5 min and the contents are maintained under inert atmosphere during all subsequent operations. The stirrer is set to 560 rpm and neat trifluoroacetic acid (TFA, 6.4 mL, 83.6 mmol, 1.5 equiv) (Note 3) is carefully added via syringe over 3 min at room temperature, whereupon the mixture becomes homogeneous.
A solution of methyl 4-hydroxyphenylacetate (1) (9.1 g, 54.8 mmol, 1.0 equiv) (Note 4) in CH3CN (20 mL), prepared in a flame-dried 50-mL round-bottomed flask, is added to the DIB solution over 3 h via syringe pump (Note 5). During the addition, the tip of the needle is immersed into the solution to a depth of 0.5 inch and the progress of the reaction is followed by TLC (Note 6). At the end of the addition (Note 7), the solution turns light brown. The mixture is transferred to a one-necked 1-L round-bottomed flask and concentrated under reduced pressure (mechanical pump vacuum, 175 mmHg) at 32 °C (rotary evaporator bath temperature). Toluene (10 mL) (Note 8) is then added and the resulting suspension is concentrated under reduced pressure to azeotropically remove residual TFA. Addition of toluene and evaporation are repeated twice again to ensure removal of essentially all of the TFA.
The resultant residue is dissolved in CH2Cl2 (100 mL) (Note 9) and silica gel (40.0 g) (Note 10) is added to the solution. The CH2Cl2 is then removed under reduced pressure (rotary evaporator, 175 mmHg, 32 °C). Residual solvent is further removed under high vacuum (0.5 mmHg). The resultant free-flowing powder is loaded onto a chromatography column (3.0 inches in diameter) that had been dry-packed with silica (160.0 g). A layer of sand (1.0 inch) was placed on top of the dry silica and a mobile phase (gradient 50%, 75%, 100% EtOAc/Hexanes, 25%, 50%, 75% MeCN/ CH2Cl2, 200 mL each) (Notes 11 and 12) is passed through the silica, followed by MeCN (400 mL) to flush out the remaining product. The fractions determined to contain product via TLC analysis (Note 6) are combined and concentrated via rotary evaporator. (Note 13) Further solvent removal under high vacuum provides the pure product (2) as a brown solid (7.7 g, 34.5 mmol, 63% yield) (Notes 14 and 15).
2. Notes
1.
(Diacetoxyiodo)benzene, also described as iodobenzene diacetate, +98%, was purchased from Alfa Aesar and was used as received.
2.
Acetonitrile, Spectroscopic grade, UltimAr®, was purchased from Macron Chemicals and was used as received.
3.
Trifluoroacetic acid, ReagentPlus 99%, was purchased from Sigma Aldrich, Inc. and was used as received.
4.
Methyl 4-hydroxyphenylacetate, 99%, was purchased from Sigma Aldrich, Inc. and was used as received.
5.
A 30 mL syringe with a diameter of 1 inch containing a 12 inch, 18-gauge needle, was used to contain a 20 mL CH
3CN solution of
1.
6.
R
f of
1 in 4:6 EtOAc/hexanes = 0.56; R
f of
2 in the same solvent system = 0; R
f of
2 in 50% MeCN/CH
2Cl
2 = 0.30.
7.
Normally, the phenol is undetectable by TLC at this point.
8.
Toluene, HPLC grade, was purchased from Fisher Scientific and was used as received.
9.
CH
2Cl
2, certified ACS, was purchased from Fisher Scientific and was used as received.
10.
Silica gel SilicaFlash® F60 (40-63 µm/230-500 mesh) was purchased from Silicycle.
11.
EtOAc, hexanes, and MeCN, certified ACS, which were used in the chromatography column, were purchased from Fisher Scientific and used as received.
12.
Fractions were collected by filling 16 x 150 mm culture tubes and the fractions containing product, R
f = 0.30, determined by TLC in 50% MeCN/CH
2Cl
2, were combined.
13.
The chromatographic procedure detailed herein was developed by the checkers. The submitters described the following alternative purification method: The brown residue obtained upon azeotropic removal of TFA with toluene was dissolved in a mixture of
Et2O (5 mL) and
CH2Cl2 (5 mL) and the solution was adsorbed onto a pad of
silica gel (55 g), covered with a layer of sand (0.25-inch), that had been prepared inside a fritted glass filter funnel (2.5-inch diameter). Water aspirator vacuum was used to draw the solution through the silica. The residue remaining in the reaction flask was dissolved with more
CH2Cl2 (10 mL) and the solution was also applied to the silica gel pad using water aspirator vacuum. The silica gel pad was first eluted (water aspirator vacuum) using 300 mL of Et
2O to remove PhI and some brown tar. The filtrate was analyzed by TLC (2.5:1, Et
2O/CH
3CN; R
f of
2 = 0.54) to ascertain that it contained little or no product; then it was discarded. Further elution (water aspirator vacuum) with a 2.5:1 (vol/vol) mixture of
Et2O/CH3CN (700 mL) retrieved the product. Vacuum concentration of the eluate afforded a semisolid brown residue, which was refiltered through a fresh pad of
silica gel (55 g) using the same procedure (complete removal of polymeric material). The residue thus obtained was taken up in
EtOAc (20 mL) and the solution was kept in a freezer at -20 °C for 5 h, whereupon the product crystallized as light orange prisms. The mother liquor was carefully decanted. The precipitate was dried by rotary evaporation under vacuum, followed by drying under high vacuum to constant mass to provide spectroscopically and microanalytically pure
2.
14.
The product is stable to storage at room temperature.
15.
Physical properties and spectral data for
2 are as follows: mp 99-100 °C (uncorrected);
1H NMR (400 MHz, acetone
-d6; the compound is poorly soluble in CDCl
3) d: 1.88 (s, 3 H), 3.01 (s, 2 H), 3.62 (s, 3 H), 6.15 (d,
J = 10.2 Hz, 2 H,), 7.18 (d,
J = 10.2 Hz, 2 H,), 7.55 (s, 1 H);
13C NMR (100 MHz, acetone
-d6) d: 23.3, 42.5, 52.0, 54.2, 129.0, 149.6, 169.9, 170.3, 185.2; IR (NaCl, cm
-1): 1739, 1668, 1536; HRMS (ESI)
m/z calculated for C
11H
13NO
4Na [M + Na]
+ 246.0742, found 246.0738. Elemental analysis (average of duplicate runs): calculated for C
11H
13NO
4: C 59.19%, H 5.87%, N 6.27%, found C 59.13%, H 5.67%, N 6.11%.
Handling and Disposal of Hazardous Chemicals
The procedures in this article are intended for use only by persons with prior training in experimental organic chemistry. All hazardous materials should be handled using the standard procedures for work with chemicals described in references such as "Prudent Practices in the Laboratory" (The National Academies Press, Washington, D.C., 2011 www.nap.edu). All chemical waste should be disposed of in accordance with local regulations. For general guidelines for the management of chemical waste, see Chapter 8 of Prudent Practices.
These procedures must be conducted at one's own risk. Organic Syntheses, Inc., its Editors, and its Board of Directors do not warrant or guarantee the safety of individuals using these procedures and hereby disclaim any liability for any injuries or damages claimed to have resulted from or related in any way to the procedures herein.
3. Discussion
The oxidative amidation of phenols
2 is a process that converts phenols
3 to dienones
5 through reaction with a hypervalent iodine reagent
3 such as (diacetoxyiodo)benzene [PhI(OAc)
2; DIB] in the presence of a suitable nitrogen nucleophile (N in
3-
5). The reaction may proceed through capture of an electrophile such as
4. The dashed semicircles in
3-
5 indicate that nucleophile and substrate may be tethered (intramolecular oxidative amidation) or may be independent (bimolecular oxidative amidation). While the process typically involves 4-substituted phenols such as
3 (
para-oxidative amidation), reaction in the
ortho mode is possible.
4 The nitrogen functionality in
5 emerges in the form of an amide (carboxamide, sulfonamide, or phosphoramide); hence the term "oxidative amidation." Intramolecular variants of the transformation include the oxidative cyclization of phenolic oxazolines,
5 sulfonamides,
4,6 and phosphoramides,
4,6 while the bimolecular reaction entails the Ritter-type interception of
4 with nitriles such as CH
3CN.
7,8 Dienones
5, especially those arising from a bimolecular reaction, are valuable building blocks for the synthesis of various nitrogenous substances.
9,10
Figure 1. The Oxidative Amidation of Phenols
In its original form,
7 the bimolecular oxidative amidation of phenols required 1,1,1,3,3,3-hexafluoroisopropanol (HFIP) as a cosolvent, and it performed well (40-70 % yield of products) on scales up to ca. 300 mg of substrate. However, the cost of HFIP and the substantial amounts of polymeric matter generated when running the reaction on a larger scale rendered the original protocol impractical and uneconomical. The present procedure, substantially that of Liang and Ciufolini,
8 is simpler and more efficient, it suppresses the need for costly HFIP, and it can be more readily scaled up. The purification of the product is expediently achieved with no aqueous workup.
Optimization of the method revealed that slow addition of a CH3CN solution of substrate to a dilute CH3CN solution of DIB and TFA is crucial to minimize polymer formation. Best results were obtained when the final concentration of substrate was around 110 mmol/L. A slight molar excess of TFA relative to DIB is necessary for efficient conversion (1.3 equiv vs DIB for small scales, 1.5 equiv vs DIB for large scales). However, larger amounts of TFA lower the yield and complicate isolation procedures.
It should be noted that the use of PhI(OCOCF3)2 (PIFA, more costly) in lieu of DIB (less costly), with or without added TFA, produces generally inferior results. This raises questions about the precise role of TFA in the reaction. First, PIFA dissolves easily in CH3CN, while DIB is poorly soluble in that solvent. Reactions run using suspensions of DIB in CH3CN proceed poorly. Addition of slightly more than 1 equivalent of TFA to a CH3CN suspension of DIB results in rapid and complete dissolution. It seems plausible that TFA promotes formation of a more soluble species such [PhI(OAc)(OCOCF3)], thereby releasing one equivalent of AcOH into the medium. Second, the foregoing mixed iodonium carboxylate is likely to possess redox properties that are intermediate between those of DIB (weaker oxidant) and PIFA (stronger oxidant). Therefore, TFA probably increases the redox potential of the oxidant to an optimal level. Third, the oxidative amidation is definitely an acid-catalyzed process: in all likelihood, a small excess of TFA provides more effective protonic catalysis than the AcOH released in the medium. Yet, as the reaction proceeds to completion, only ca. 1 equivalent of TFA is liberated, as opposed to the 2 equivalents released by PIFA. This minimizes undesirable acid-promoted side reactions. Fourth, the Ritter-type capture of an electrophile arising through oxidative activation of the phenol would lead to nitrilium ion 6, which presumably must combine with a nucleophile. Undoubtedly, AcOH is more nucleophilic than TFA. If reactive intermediate 6 were form in a medium already containing AcOH, its conversion to 7, and thence to 8, would be more efficient.
Figure 2. Possible Mechanism of the Bimolecular Oxidative Amidation of Phenols
The procedure described herein appears to have substantial scope with respect to the phenolic substrate. Representative examples are provided in Table 1.
8
Table 1. Representative Examples of Bimolecular Oxidative Amidation of Phenols
Appendix
Chemical Abstracts Nomenclature (Collective Index Number);
(Registry Number)
(Diacetoxy)iodobenzene: iodobenzene 1,1-diacetate, iodosobenzene 1,1-diacetate; (3240-34-4)
Trifluoroacetic acid; (76-05-1)
Methyl 4-hydroxyphenylacetate; (14199-15-6)
1,1,1,3,3,3-Hexafluoroisopropanol: hexafluoroisopropanol (920-66-1)
2,5-Cyclohexadiene-1-acetic acid, 1-(acetylamino)-4-oxo, methyl ester (837373-69-0)
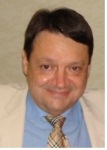 |
Marco A. Ciufolini [B.S., Spring Hill College, Mobile, AL (1978), Ph.D., University of Michigan (1981, M. Koreeda), postdoc, Yale University (1982-84, S. Danishefsky)] has held academic positions at Rice University (Houston, TX, 1984-1997), the Ecole Supérieure de Chimie, Physique et Electronique de Lyon and the University of Lyon (Lyon, France, 1998-2004), and the University of British Columbia at Vancouver (2004-present), where he is currently the Canada Research Chair in Synthetic Organic Chemistry. His work focuses on the development of new synthetic methods and their application to the synthesis of nitrogenous substances. |
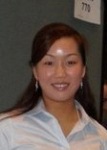 |
Jaclyn Chau [B.Sc., University of British Columbia, Vancouver, BC (2009)] joined the research group of Professor Marco A. Ciufolini in 2008 at the University of British Columbia. Her current research centers on the total synthesis of nitrogenous natural products. |
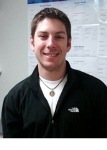 |
Aaron Bedermann was born in Madison, Wisconsin. He received his BS in Chemistry from the University of Wisconsin, where he performed undergraduate research under the supervision of Professor Richard Hsung. He is currently pursuing graduate research at Colorado State University under the guidance of Professor John L. Wood. |
Copyright © 1921-, Organic Syntheses, Inc. All Rights Reserved