Org. Synth. 2012, 89, 409-419
DOI: 10.15227/orgsyn.089.0409
Discussion Addendum for:
[N-Hydroxy-4-(p-chlorophenyl)thiazole-2(3H)-thione]
Submitted by Christine Schur, Irina Kempter, and Jens Hartung
*1
.
Discussion
O-Alkylation of
N-hydroxythiazole-2(3H)-thiones
(TTOHs) provides
N-alkoxy derivatives, which are valuable alkoxyl radical precursors for photobiological, mechanistic, and synthetic purposes.
2,3,4 Chemical reactivity of the
N-alkoxythiazole-2(3H)-thiones (TTORs) is controlled by the thiohydroxamic acid functional group that is embedded in a cross-conjugated heterocyclic π-electron system.
5,67 Breaking of the thiohydroxamate nitrogen-oxygen bond in TTORs allows the heterocyclic fragment to gain aromatic stabilization, which is the reason for the molecules to liberate oxygen-centered radicals under comparatively mild conditions.
Systematic exploration of
O-alkyl thiohydroxamate-based
O-radical precursors started with the
N-alkoxypyridine-2(1
H)-thiones.
8,9,10 Experimental difficulties associated with synthesis and storage of the reagents stimulated development of the
N-alkoxy-4-(p-chlorophenyl)thiazole-2(3H)-thiones (CPTTORs).
The latter compounds have shelf-lives from months to years,
11,12 but selectively liberate oxygen radicals if photochemically or thermally excited.
4 Problems remaining unsolved in CPTTOR-chemistry, such as synthesis of tertiary
O-alkyl derivatives, were successfully addressed by introducing
N-hydroxy-5-(p-methoxyphenyl)-4-methylthiazole-2(3H)-thione (MAnTTOH) as next generation alkoxyl radical progenitor.
3,13,14
The Chemistry of N-Alkoxy-4-(p-chlorophenyl)thiazole-2(3H)-thiones
CPTTOH is an acid of similar strength to acetic acid and forms primary and secondary
O-esters in 50-70% yield, if converted into the derived tetraalkylammonium salt and treated with a hard alkylating reagent.
The largest body of chemical reactivity data compiled for the CPTTORs relate to photochemically or thermally initiated reactions with typical mediators for conducting radical chain reactions, such as tributylstannane, tris(trimethylsilyl)silane,
O-ethyl cysteine, or
bromotrichloromethane.
5,15,16,17 In a supplementary project, the propensity of
O-acyl- and
O-alkyl-derivatives of CPTTOH to crystallize was used to study the until then unexplored solid state chemistry of the thiohydroxamates.
The results from this study show that the molecules tolerate a significant degree of steric congestion at the thiohydroxamate oxygen, because strain effects are absorbed by structural changes across the whole thiohydroxamte group.
18
In addition to radical reactions and solid state chemistry, new ground state reactivity of CPTTORs was discovered, such as a shift of the methyl group from oxygen to sulfur, fragmentation of secondary benzylic esters leading a thiolactam and a ketone, and 4-pentenoxy rearrangements occuring specifically in the solid state (Figure 1).
19,20
Figure 1.
Background reactivity of neat CPTTORs in the solid state.
5-Substituted N-Hydroxy-4-methylthiazole-2(3H)-thiones
From a structure-reactivity study it became apparent that a substituent attached in position 5 shifts electronic absorptions of the thiazole-2(3
H)-thione nucleus stronger than the same group does in position 4.
Synthesis of 5-substituted
N-hydroxy-4-methylthiazole-2(3H)-thiones (Figure 2) starts from constitutionally dissymmetric ketones.
Arylpropanones that are not commercially available can be prepared from underlying arylcarbaldehydes and nitroethane via intermediate β-nitrostyrenes.
Hydrolysis of nitrostyrenes in acidic aqueous solutions containing iron-powder furnishes the required ketones, which are chlorinated by sulfuryl chloride at the higher substituted α-carbon.
Methods of
O-ethyl xanthogenate- and oxime-synthesis follow the protocol of the original CPTTOH-synthesis (Figure 2).
For the final step, potassium hydroxide in an equimolar volume of dichloromethane and water is the more efficient reagent to mediate the thiazolethione ring closure compared to anhydrous zinc chloride in diethyl ether, which is the recommended reagent for the final step of CPTTOH-synthesis.
3
Figure 2.
Synthesis of 5-substituted
N-hydroxy-4-methylthiazole-2(3
H)-thiones.
3
N-Alkoxy-4-methylthiazole-2(3H)-thiones
N-Hydroxy-5-(p-methoxyphenyl)-4-methylthiazole-2(3H)-thione (MAnTTOH) was developed as successor of CPTTOR, to improve photochemical properties of alkoxyl radical progenitors, raise yields of
O-alkylation products, and prepare
tert-
O-alkyl thiohydroxamates which until then were not available in yields higher than 5%.
MAnTTORs show a longest wavelength of absorption at around 334 nm that gives some of the compounds a yellow color and allows photochemical excitation with visible light or 350 nm-light from a Rayonet
®-photoreactor.
MAnTTOH-derived thiohydroxamate salts show an unusual propensity to form products of
O-alkylation (MAnTTORs), if treated with alkyl tosylates, alkyl chlorides, or even alkyl iodides (top graphic of Figure 3).
20 The majority of MAnTTORs are solids that crystallize by adding an excess of methanol to crude reaction mixtures.
Alkylation at sulfur, which consumes the major fraction of alkylation reagent in
N-alkoxypyridine-2(1
H)-thione-synthesis, is restricted to comparably few instances, such the reaction between the MAnTTOH-tetraethylammonium salt and methyl iodide (46%; bottom graphic of Figure 3).
Figure 3.
Preparation of MAnTTORs (top and table; An =
p-anisyl) and selective
S-alkylation of a MAnTTOH-derived tetraethyl-ammonium salt (bottom).
20
Alternative methods for thiohydroxamate
O-alkylation are the Mitsunobu-reaction, cyclic sulfate-opening, and
O-alkyl isourea-chemistry (Figure 4).
The former two methods proceed via an inversion of configuration at the attacked carbon,
21 and the latter is the only method available so far to prepare tertiary
O-alkyl thiohydroxamates.
22
Figure 4.
Synthesis of
O-alkyl thiohydroxamates from an alcohol, cyclic sulfate, or an isourea.
21,22
Scope of the Thiohydroxamate Method
The advantage of
N-alkoxythiazole-2(3
H)-thiones compared to alternative alkoxyl radical precursors is their ability to liberate alkoxyl radicals in a propagating step of a chain reaction.
Nitrogen-oxygen bond homolysis thereby occurs after addition of a chain propagating radical, such as a carbon-, silicon-, or tin-radical, to the thione-sulfur (Figure 5).
14 Alkoxyl radicals are highly reactive intermediates, their selectivities are, however, predictable in a straightforward manner on the basis of kinetic and thermochemical data.
Additions and hydrogen-atom abstractions, two of the main alkoxyl radical reactions, are irreversible and therefore controlled by kinetic effects (Figure 6, top and center).
Selectivity of β-carbon-carbon fragmentation for synthesis of carbonyl compounds (Figure 6, bottom), and alkoxyl radical rearrangements are guided by radical stabilizing effects and therefore are thermodynamically controlled.
2,20,23
Figure 5.
Radical chain mechanism for synthesis of target compounds according to the thiohydroxamate method, exemplified by the use of BrCCl
3 as mediator (R = alkyl, phenyl, acyloxy; R' = hydrogen, alkyl, phenyl).
20,22
Figure 6.
Application of homolytic substitution, addition, and β-carbon-carbon bond fragmentation of alkoxyl radicals in synthesis.
24
Since the radical oxygen is electrophilic and the hydroxyl oxygen nucleophilic, additions of alkoxyl radicals and alcohols to polar carbon-carbon double bonds occur with complementary selectivity (Figure 7).
7,25,26,27
Figure 7. Examples showing complementary selectivity in bromocyclizations of alkenoxyl radicals (top) and alkenols (bottom: VOL(OEt) = 2-[(2-oxidophenyl)iminomethyl] (ethanolato) oxidovanadium(V); py·HBr = pyridinium hydrobromide; TBHP =
tert-butylhydroperoxide}.
25,27
Alkoxyl radical generation from
N-alkoxythiazolethiones occurs under pH-neutral, non-oxidative conditions and therefore may be used for transformations that are not attainable by other alkoxyl radical-generating methods.
Substituted 5-hexenyloxyl radicals, for example, cyclize onto π-bonds having two methyl groups attached at the terminal alkene carbon.
The reaction stereoselectively provides tetrahydropyranylmethyl radicals, which are trapped by bromotrichlormethane to furnish bromocyclized products (Figure 8).
28,29 Polar bromocyclization of the underlying 5-hexenols provide oxepans as major products.
Figure 8.
Synthesis of tetrahydropyrans from MAnTTORs via underlying 5-hexenoxyl radical cyclization.
The thiohydroxamate method also applies to prepare vicinal bromohydrin ethers from intermolecular alkoxyl radical addition to alkenes (Figure 9).
O-Alkyl thiohydroxamates thus were used to synthesize norbornene-derived vicinal bromohydrin ethers, which have interesting biological properties but are difficult to prepare from ionic reactions due to complications imposed by non-classical carbocation formation.
22,30
Figure 9.
Synthesis of β-bromoethers from intermolecular alkoxyl radical additions.
Besides adding benefits to organic synthesis, MAnTTORs are excellent tools for conducting kinetic studies on alkoxyl radical elementary reactions.
22 Kinetic data are the basis for application of alkoxyl radicals in synthesis of ethers , for complementing selectivity that for more than a century has been restricted to nucleophilic carbon-oxygen bond formation.
Appendix
Chemical Abstracts Nomenclature (Collective Index Number);
(Registry Number)
bromotrichloromethane (75-62-7)
N-HYDROXY-4-(p-CHLOROPHENYL)THIAZOLE-2(3H)-THIONE (105922-93-8)
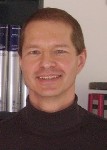 |
Jens Hartung was born in Offenbach/Main, Germany in 1961.
He took his diploma degree in 1987 with Klaus Hafner and his Ph.D.
with Bernd Giese in 1990 at the Technische Hochschule Darmstadt, Germany.
That same year, he moved to the MIT to spent a postdoctoral year with K.
Barry Sharpless.
In 1992 he joined the cardiovascular division of Hoechst AG (today Sanofi-Aventis).
In 1994 he moved as lecturer to the Bayerische Julius-Maximilians-Universität Würzburg, where he completed his habilitation in 1998.
Since 2003 he is full professor of organic chemistry at the Technische Universität Kaiserslautern.
His research interests include the chemistry of reactive intermediates, in particular oxygen-centered radicals, transition metal-catalyzed oxidations, the chemistry of vanadium-dependent bromoperoxidases, static and dynamic stereochemistry, synthesis of heterocycles and marine natural products.
|
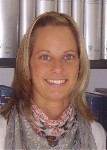 |
Christine Schur was born in Karassu, Kasachstan in 1983.
She received a Diploma-degree in 2008 from the Technische Hochschule Kaiserslautern studying selectivity in inter- and intramolecular alkoxyl radical reactions, working with Jens Hartung.
She continues this project as part of her Ph.D.-thesis.
|
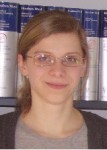 |
Irina Kempter was born in Neustadt/Weinstraβe, Germany in 1984.
She received a Diploma-degree in 2008 from the Technische Hochschule Kaiserslautern studying stereoselective O-alkyl thiohydroxamate synthesis, working with Jens Hartung.
Her research interests in a new project as part of her Ph.D.-thesis are associated with investigation of polar effects in alkoxyl radical chemistry.
|
Copyright © 1921-, Organic Syntheses, Inc. All Rights Reserved