Org. Synth. 2009, 86, 333
DOI: 10.15227/orgsyn.086.0333
SYNTHESIS OF 4,5-DIMETHYL-1,3-DITHIOL-2-ONE
Submitted by Perumalreddy Chandrasekaran and James P. Donahue
1.
Checked by Daniel M. Bowles and John A. Ragan.
2.
1. Procedure
A. O-Isopropyl S-3-Oxobutan-2-yl Dithiocarbonate (1). A 1-L, 3-necked flask, equipped with a 125-mL pressure equalizing addition funnel and nitrogen inlet is oven-dried, assembled hot, and cooled to ambient temperature under nitrogen vented to an external bubbler. The center neck is equipped with an overhead stirrer and the third neck is fitted with an internal temperature probe. The apparatus is cooled to ambient temperature and is charged with anhydrous K2CO3 (65 g, 470 mmol, 1.4 equiv) (Note 1) followed by freshly distilled 2-butanone (500 mL, 403 g, 5.58 mol, 16 equiv) (Note 2). The resulting suspension is cooled to below -75 °C with an acetone/dry ice bath, and a solution of bromine (17.7 mL, 55.2 g, 0.345 mol, 1.0 equiv) (Note 3) in 100 mL CH2Cl2 (Note 4) is added dropwise via the addition funnel over 30 min, maintaining the internal pot temperature below -70 °C (Note 15, photograph A). The resulting orange-colored reaction mixture is removed from the cold bath and is allowed to warm to 18 °C over 4 h. During this time, the color of the mixture changes from orange to colorless. The reaction mixture is stirred for an additional 4 h at ambient temperature. Potassium O-isopropylxanthate (Note 5) (60.4 g, 0.346 mol, 1.0 equiv) is then charged via a solids addition funnel in a single portion under nitrogen.
The resulting reaction mixture is heated to 60 °C (internal temperature) with a heating mantle for 6 h. The progress of the reaction can be conveniently monitored by silica gel TLC (9:1 heptanes/EtOAc; potassium O-isopropylxanthate Rf=0.0; desired product Rf=0.25). The heterogeneous mixture is cooled to 18 °C and then vacuum filtered in the open air through a packed 2 inch celite pad on a medium porosity, 3.5" diameter glass frit. The filter contents are washed with CH2Cl2 (3 × 100 mL), and the combined filtrates are concentrated to dryness at 50 °C and 20 mmHg in a 1-L, round-bottomed flask on a rotary evaporator to afford 59.6-61.7 g (0.289-0.299 mol, 83-86% yield based on potassium O-isopropylxanthate) of O-isopropyl S-3-oxobutan-2-yl dithiocarbonate 1 as an orange oil that can be carried forward without further purification. Analytically pure material is obtained by vacuum distillation from a 150-mL, single-necked, round-bottomed flask with a 10-cm Vigreaux column at 79-82 °C and 0.1 mmHg, which yields 48.9-50.1 g (0.237-0.243 mol, 68-70%) of 1 as a pale yellow, pungent smelling oil (Notes 6 and 7).
B. 4,5-Dimethyl-1,3-dithiol-2-one (2). A 250-mL, 3-necked, round-bottomed flask equipped with a magnetic stirring bar, 250-mL pressure-equalizing addition funnel, internal temperature probe and glass stopper is charged with previously distilled O-isopropyl S-3-oxobutan-2-yl dithiocarbonate 1 (53.0 g, 0.257 mol), and the neat oil is cooled to 0 °C. Ice-cooled 15 M aqueous H2SO4 (100 mL, 1.5 mol, Note 8) is added dropwise via addition funnel over 30 min, maintaining the pot temperature below 10 ºC. The reaction mixture is maintained between 0 °C and 10 °C for 1 h, warmed to room temperature, and then stirred for an additional hour. During this time, the reaction mixture darkens progressively to a brown color. Reaction progress is conveniently monitored by silica gel TLC analysis (9:1 heptane/EtOAc; SM Rf=0.25; product Rf=0.35). The reaction is quenched by slowly pouring the contents over 500 mL of ice in 10 mL portions over a 15 min period. The resulting two-phase aqueous-organic mixture is transferred to a 1-L separatory funnel and is extracted with Et2O (3 × 200 mL) (Note 9). The combined Et2O extracts are dried by stirring over MgSO4 (75 g) for 2 h. The MgSO4 is removed by vacuum filtration on a coarse, sintered glass filter, and the cake is washed with 2 × 200 mL Et2O. The filtrates are concentrated on a rotary evaporator (25 °C, 20 mmHg) to produce a crude, dark, waxy solid that is purified by short-path vacuum distillation from a 100-mL, single-necked, round-bottomed flask (Note 10 and Note 15, photograph B) at 60-64 °C and 0.1 mmHg to afford 31.6-32.5 g (0.216-0.222 mol, 84-87%) of 4,5-dimethyl-1,3-dithiol-2-one as a pale yellow oil that solidifies upon standing (Notes 11 and 13).
Highly crystalline material is prepared by dissolving 5.0 g of freshly distilled 4,5-dimethyl-1,3-dithiol-2-one in 25 mL warm hexanes followed by cooling to -25 °C to afford 4.10 g (82%) of off-white needle crystals that are isolated by decanting the mother liquor and drying at 18 °C in open air. Crystallization of 5.0 g crude (non-distilled) 2 in the same manner produces a lower recovery (2.1 g, 42%) of brown needles that are relatively clean by 1H and 13C NMR (Note 15, photograph C). Compound 2 is stable to air, moisture and light and is soluble in common organic solvents such as pentane, hexane, diethyl ether, dichloromethane, benzene, toluene, acetonitrile and methanol (Note 14).
2. Notes
1.
Anhydrous potassium carbonate (ReagentPlus grade, 99%) from Sigma-Aldrich was used as received. The use of K
2CO
3 is necessary to scavenge the HBr generated during the bromination reaction. In one instance, the checkers noted a poor yield (21%) resulting from using older, 'wet' potassium carbonate.
2.
Reagent grade 2-butanone (methyl ethyl ketone) was obtained from Sigma Aldrich (99+%) and distilled from CaSO
4 at ambient pressure using a fractionating column. A forerun is set aside. The 2-butanone used in the procedure is collected when the distillation is steady at ~80 °C. The 2-butanone is used both as solvent and substrate in this reaction. The use of smaller volumes of 2-butanone results in heterogeneous mixtures that are difficult to stir.
3.
Reagent grade bromine from Sigma Aldrich (99.5%) was used as received.
4.
Reagent grade dichloromethane from JT Baker was used without additional purification.
5.
Potassium
O-isopropylxanthate is available commercially from a variety of suppliers (e.g. $44.10 / 25 g from TCI America, used as received). The checkers noted that one-portion addition of this material resulted in an average exotherm of 20 °C at this scale. Care should be taken on larger scales to insure proper jacket cooling is available.
6.
Pure
O-isopropyl
S-3-oxobutan-2-yl dithiocarbonate may be stored in the open air at ambient temperature without noticeable decomposition for a period of months. The material obtained after rotary evaporation of the CH
2Cl
2 and 2-butanone solvent is generally of sufficient purity for the next step. This determination is made on the basis of
1H NMR spectroscopy and by the observation of only slightly diminished overall yields using undistilled
1.
7.
The spectroscopic and analytical properties of
O-isopropyl
S-3-oxobutan-2-yl dithiocarbonate (
1) are as follows: bp: 79-82 °C at 0.1 mmHg. R
f: 0.25 on silica (9:1 heptane:EtOAc), visualization by UV-vis.
1H NMR
pdf (CDCl
3, 400 MHz, 25 ºC) δ: 1.359 (d,
J = 6.4 Hz, 3 H), 1.363 (d,
J = 6.4 Hz, 3 H), 1.43 (d,
J = 7.6 Hz, 3 H), 2.28 (s, 3 H), 4.34 (q, J = 7.2 Hz, 1 H), 5.69 (sep,
J = 6.4 Hz, 1 H).
13C NMR
pdf (CDCl
3, 100 MHz, 25 ºC) δ: 15.0, 20.69, 20.74, 26.9, 53.1, 78.3, 203.9, 210.8. FTIR (neat oil, ATR, cm
-1): 2981, 1717, 1386, 1374, 1356, 1242, 1157, 1143, 1091, 1038, 900, 631, 537. CI-MS:
m/z 207 ([M + H]
+, 46), 165 (M -
iPr, 77), 147 (M - O
iPr, 26), 105 (100). HRMS: (ESI+)
m/z: 207.05080 [calcd for C
8H
15O
2S
2 (M+H) 207.05090]. Anal. Calcd. for C
8H
14O
2S
2: C, 46.57; H, 6.84; S, 31.08. Found: C, 46.53; H, 6.80; S, 31.15.
8.
An 80 mL volume of concentrated H
2SO
4 is diluted to 100 mL by slowly pouring it into a 20 mL volume of ice cold water, resulting in a 15
M solution. The checkers noted a poor yield (34%) when sulfuric acid was added too quickly to the pot mixture without proper cooling.
9.
The aqueous acidic phase should be brought to a neutral pH with saturated aqueous K
2CO
3 and transferred to an aqueous waste storage bottle.
10.
The condenser of the short-path distillation apparatus should not be cooled with cold water. During the course of the vacuum distillation, 4,5-dimethyl-1,3-dithiol-2-one solidifies within the distillation condenser and requires mild, continuous heating with a heat gun to ensure that it remains melted until collected in the distillation receiver. Alternatively, the checkers found that circulation of warm (55 ºC) fluid through the short-path distillation head is highly effective.
11.
The submitters obtained an additional 2.8% of product by chromatographic purification of the dark distillation pot residue as follows. Following the distillation of 4,5-dimethyl-1,3-dithiol-2-one obtained from the cyclization of 62.3 g (0.302 mol) of undistilled
O-isopropyl S-3-oxobutan-2-yl dithiocarbonate, an additional 1.25 g (8.53 mmol, 2.8% yield) of product is recovered. The column is packed as a silica slurry
(Note 12) in hexanes (1 in diameter × 10 in height) and is loaded by evaporation of a concentrated CH
2Cl
2 solution of the distillation pot residues onto 10 g silica followed by transferal of this dry coated silica directly onto the column. The column is then eluted with a gravity drip. The 4,5-dimethyl-1,3-dithiol-2-one readily and completely resolves from the other components of the mixture as the leading band and is completely eluted within a 150 mL volume of hexanes after loading.
12.
The silica used for the column was 70-230 micron and was obtained from EMD Chemicals (Merck), Gibbstown NJ, Catalog Number 7734-3.
13.
The checkers found that using undistilled
1 in Step B (61.7 g, 0.299 mol) provided 25.9 g of
2 (0.177 mol, 59% yield).
14.
Spectroscopic and analytical data for 4,5-dimethyl-1,3-dithiol-2-one are as follows: mp: 46-48 ºC. bp: 59-64 °C at 0.1 mmHg. R
f: 0.35 on silica (9:1 heptane:EtOAc), visualization by UV-vis.
1H NMR
pdf (CDCl
3, 400 MHz, 25 ºC) δ: 2.13 (s).
13C NMR
pdf (CDCl
3, 100 MHz, 25 ºC) δ: 13.7, 122.8, 192.1. FTIR (neat oil, ATR, cm
-1): 3243, 2942, 2851, 1753, 1650, 1596, 1433, 1373, 1189, 1092, 1017, 941, 866, 756. CI-MS:
m/z 147 ([M + H]
+, 100). HRMS: [M + H] calcd for C
5H
7O
1S
2: 146.99329. Found: 146.99333. Anal. Calcd. for C
5H
6OS
2: C, 41.07; H, 4.14; S, 43.86. Found: C, 41.11; H, 4.04; S, 44.75.
15.
Photographs of the procedure:
(A) Step A during bromine addition.
(B) Step B product distillation
(C) Comparison of Step B product (2) obtained by recrystallization of distilled (left two samples) or undistilled (right two samples) material
Scheme 1
3. Discussion
The 1,3-dithiol-2-ones constitute a class of molecules with demonstrated synthetic utility for the synthesis of tetrathiafulvalenes and related materials (Scheme 1). Oligo- and polytetrathiafulvalenes have been subjected to intense investigation for a broad variety of applications ranging from conducting organic materials
3-9 to solar energy conversion systems
10 to sensors
3,11 and switching devices.
7,11 A key reaction for the synthesis of many kinds of elaborated tetrathiafulvalenes is the phosphite mediated coupling of 1,3-dithiol-2-chalcogenones to form the tetrathio-substituted olefinic bond. First reported by Corey
12 and later extended by Miles,
13 this reaction type has become ubiquitous for the synthesis of all manner of tetrathiafulvalenes. The 1,3-dithiol-2-ones are also useful synthetically as protected forms of dithiolene ligands that are readily unmasked by simple base hydrolysis (Scheme 1).
14-15 The rich electrochemical and photophysical behavior associated with metal dithiolene complexes has also stimulated a large body of research aimed at applications such as superconducting materials,
16 sensing devices,
17 photocatalysts,
18 and reversibly bleachable dyes for Q-switching in near IR lasers.
19
Scheme 2
One of the more simple, symmetrically substituted 1,3-dithiol-2-ones is the 4,5-dimethyl form. Despite the amenability of this compound to further derivatization, e.g., by bromination of the methyl groups and subsequent nucleophilic substitution,
6,20 its use has been more limited than would be expected. A considerable number of molybdenum and tungsten dithiolene complexes with the dimethyl-substituted ligand have been reported as structural and functional analogues of the catalytic sites of molybdo-and tungstoenzymes,
21-22 but in all instances the ligand is derived ultimately from 3-hydroxy-2-butanone/P
4S
10 rather than through a more direct approach proceeding through 4,5-dimethyl-1,3-dithiole-2-one. A possible reason for the surprisingly little use that has been made of 4,5-dimethyl-1,3-dithiole-2-one as a protected dithiolene ligand is an underappreciation of the ease by which it may be prepared in large, multigram quantities.
The preparation of this useful molecule can be accomplished by several distinct routes (Scheme 2). One approach involves the radical-initiated reaction of diisopropyl xanthogen disulfide with 2-butyne to form the 1,3-dithiol-2-one directly (Path
A).
23 A related synthesis describes the palladium-mediated coupling of bis(triisopylsilyl)disulfide with 2-butyne to yield 2,3-bis(triisopropylsilanylsulfanyl)but-2-ene, which is subsequently treated with phenyl chlorothiolformate and TBAF to form the 1,3-dithiol-2-one (Path
B).
24 Another method begins with the reaction between 3-bromo-2-butanone and an
O-alkylxanthate salt to afford an
O-alkyl
S-3-oxobutan-2-yl dithiocarbonate intermediate (Path
C) that is then subjected to acid-catalyzed ring closure.
20,25-26 The 4,5-dimethyl-1,3-dithiol-2-one product is also available via mercuric acetate mediated chalcogen exchange
27 from the corresponding trithiocarbonate (Path
D).
28 The route to 4,5-dimethyl-1,3-dithiol-2-one that begins with 3-bromo-2-butanone and potassium
O-isopropylxanthate avoids the use of 2-butyne and its associated flammability and expense. While this reaction sequence has been previously reported,
20,25-26 we describe procedural modifications that enable the cost effective synthesis of 4,5-dimethyl-1,3-dithiol-2-one on a scale of tens of grams. In contrast, the largest reported scale on which it has previously been reported is seven grams.
27
The first point we emphasize is that the a-bromo ketone that is condensed with
O-isopropylxanthate can be generated
in situ from 2-butanone/Br
2 and used immediately. The 2-butanone can in fact be used in excess as both solvent and reagent. Previously published procedures have described the use of a-bromo ketone purchased from commercial sources or have implied a need to prepare and isolate it in a discrete step. The ease and selectivity with which 2-butanone is brominated in the 3-position and the intrinsic reactivity of the resulting a-bromoketone render
in situ generation and immediate use a greatly preferable approach. The second procedural improvement we note for the synthesis of 4,5-dimethyl-1,3-dithiol-2-one is that, although a solid at ambient temperature and pressure, it has a low melting point and is sufficiently volatile at reduced pressure as to be amenable to vacuum distillation. A simple short-path distillation can yield a large volume of pure 4,5-dimethyl-1,3-dithiol-2-one without the need to resort to column chromatography as a purification method, as has been reported earlier.
20 Together, these improvements should make this useful molecule much more accessible than has heretofore been recognized.
Appendix
Chemical Abstracts Nomenclature (Collective Index Number);
(Registry Number)
O-Isopropyl S-3-Oxobutan-2-yl Dithiocarbonate: Carbonodithioic acid, O-(1-methylethyl) S-(1-methyl-2-oxopropyl) ester; (958649-73-5)
2-Butanone; (78-93-3)
Potassium O-isopropylxanthate: Carbonodithioic acid, O-(1-methylethyl) ester, potassium salt (1:1); (140-92-1)
4,5-Dimethyl-1,3-dithiol-2-one; (49675-88-9)
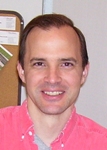 |
James P. Donahue was born in 1968 in Mishawaka, IN. He graduated in 1991 from M.I.T. with a B.S. in chemistry and joined the laboratory of Richard H. Holm of Harvard University as an NDSEG predoctoral fellow. His doctoral work concerned synthetic analogues of the active sites of molybdo- and tungstoenzymes. In 1998, he received his Ph.D. and moved to Texas A&M University to do postdoctoral work with F. A. Cotton with the support of an NIH postdoctoral fellowship. In 2004, he accepted a position at Tulane University in New Orleans, where he is currently an assistant professor of chemistry. |
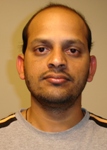 |
Chandrasekaran was born in 1979 in Kakkanur, Tamil Nadu, India. He obtained his Bachelors degree in chemistry from Sacred Heart College (Tirupattur) and Masters from Pachaiyappa's College at Chennai. He then moved to Indian Institute of Technology Bombay for his Ph.D. studies under the guidance of Prof. Maravanji S. Balakrishna. His doctoral research was focused on organometallic chemistry of cyclic phosphorus-nitrogen compounds. He is currently a post-doctoral researcher with Prof. James P. Donahue at Tulane University. |
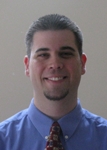 |
Daniel M. Bowles was born in Charleston, West Virginia and received a B.S. in Chemistry from West Virginia Wesleyan College in 1996. He earned a Ph.D. in Organic Chemistry from the University of Kentucky under Dr. John Anthony in 2000 for his work on multi-step synthesis of a series of polycyclic aromatic oligomers. He joined C. Edgar Cook at RTI in Research Triangle Park, North Carolina for postdoctoral study in steroid research, then took a position with Pfizer Chemical Research and Development in Holland, Michigan in late 2001. He moved with Pfizer to the Ann Arbor site in 2003, and again to Groton, CT in 2007, where he currently works as a Principal Scientist in the RAPI (early candidate development) group. |
Copyright © 1921-, Organic Syntheses, Inc. All Rights Reserved