Org. Synth. 2007, 84, 22
DOI: 10.15227/orgsyn.084.0022
SYNTHESIS OF ACYLSILANES FROM MORPHOLINE AMIDES. SYNTHESIS OF 1-(DIMETHYL(PHENYL)SILYL)PROPAN-1-ONE
[Dimethyl(1-oxopropyl)phenylsilane]
Submitted by Robert B. Lettan II, Benjamin C. Milgram, and Karl A. Scheidt
1
.
Checked by Scott E. Denmark and Ramil Y. Baiazitov.
1. Procedure
A. 1-Morpholinopropan-1-one. A flame-dried, 1-L, three-necked, round-bottomed flask, equipped with a magnetic stirring bar, 50-mL addition funnel (capped with a rubber septum), a nitrogen inlet and Teflon-coated thermocouple (Note 1) is charged with 32.9 mL (32.9 g, 378 mmol, 1.1 equiv) of morpholine (Note 2), which is added by syringe to the addition funnel with the stopcock open. Methylene chloride (175 mL) (Note 3) is added by syringe through the same septum and is used to rinse the addition funnel. The stopcock is closed and solution is placed under a positive pressure of nitrogen from a manifold. While stirring the solution, the flask and its contents are cooled in a NaCl/ice bath (internal temperature −8 °C). To the stirred solution is added propanoyl chloride (15.0 mL, 15.9 g, 172 mmol) (Note 4) through the addition funnel over 20 min (Note 5). Following the addition, the mixture is allowed to warm to ambient temperature. The mixture is stirred for 23 h at ambient temperature (Note 6). Then the solution is diluted with 70 mL of methylene chloride and transferred to a 1-L separatory funnel. The mixture is subsequently washed with 1N HCl (2 × 50 mL), and a mixture of saturated aqueous sodium bicarbonate (25 mL) and brine (50 mL) in a 1-L separatory funnel. The organic phase is dried over 10 g of anhydrous magnesium sulfate, filtered through glass wool, and concentrated by rotary evaporation (10 mmHg) to provide 27.4 g of a colorless liquid. The product is distilled with a short path distillation head (50 mm Vigreaux column) (oil bath temperature 110–120 °C) to afford 21.3 g (86%) of 1-morpholinopropan-1-one as a clear liquid (bp 83–86 °C, 1.1 mmHg) (Notes 7, 8).
B.
Dimethylphenysilyllithium. This reagent is prepared according to the procedure of Fleming and coworkers.
2 A flame-dried,
300-mL, two-necked, Schlenk flask equipped with a magnetic stirring bar, nitrogen inlet and a rubber septum is charged with 33 cm (45 mg/cm, 1.49 g, 214 mmol, 1.5 equiv) of lithium wire
(Note 9) and is filled with nitrogen.
Tetrahydrofuran (60 mL, Note 10) is added by syringe through the septum. To the suspension of lithium wire is added
chlorodimethylphenylsilane (12.0 mL, 71.5 mmol) (Note 11) by syringe at ambient temperature with stirring
(Note 12). Following the addition of chlorodimethylphenylsilane, the reaction flask is tightly sealed and then transferred to a −15 to −20 °C freezer and is allowed to stand for 59 h. The resulting, purple solution of dimethylphenylsilyllithium in THF is titrated to 0.97 M against phenolphthalein with 0.1 N HCl
(Note 13).
C. 1-(Dimethyl(phenyl)silyl)propan-1-one. A flame-dried, 250-mL, 3-necked, round-bottomed flask, equipped with a magnetic stirring bar, a nitrogen inlet, a Teflon-coated thermocouple and a rubber septum, is charged with of 1-morpholinopropan-1-one (6.0 g, 41.9 mmol) by syringe under a nitrogen atmosphere from a manifold. To the flask is added 45 mL of THF (Note 10) by syringe. The solution is then cooled to −74 °C (internal) with a dry ice/acetone bath and dimethylphenylsilyllithium in THF (0.97 M solution, 52 mL, 50.4 mmol, 1.2 equiv) is added dropwise by syringe over 8 min such that the internal temperature never rises above −60 °C. The purple reaction mixture is stirred for 70 min at −75 °C (Note 14), quenched with 100 mL of saturated aqueous ammonium chloride solution, and then is allowed to warm to ambient temperature. The mixture is diluted with 40 mL of water in a 1-L separatory funnel and is extracted with hexanes (150 mL) and ethyl acetate (2 × 50 mL). The combined organic extracts are washed with saturated aqueous ammonium chloride (50 mL) and brine (100 mL), then are dried over 11 g of anhydrous magnesium sulfate, filtered through glass wool, and concentrated by rotary evaporation under vacuum (10 mm Hg). The residue is further dried by azeotropic evaporation with hexanes (2 × 50 mL, 10 mmHg) to afford 10.0 g of a light-yellow oil. The product is purified by chromatography on silica gel (50 mm column diameter, 150 g silica gel, packed with precooled hexanes (−15 °C)) using a gradient of precooled solvents (hexanes/CH2Cl2: 1/0 (350 mL), 10/1 (500 mL), 5/1 (550 mL), 1/1 (450 mL), 0/1 (250 mL), 60 mL fractions) to provide the acylsilane (5.30 g, 66%) as a light yellow oil and 0.63 g (8%) of a less pure fraction. The larger portion was distilled using a 65-mm Vigreaux column (oil bath, 130 °C) to afford 4.73 g (59%) of 1-(dimethyl(phenyl)silyl)propan-1-one as a light-yellow oil (bp 69–72 °C at 0.94 mmHg) (Notes 15, 16, 17).
2. Notes
1.
A PFA-coated thermocouple probe, Type K (Omega Engineering, Inc.) was inserted through a teflon thermometer adapter sealed with an O-ring.
2.
Morpholine (99+%) was purchased from Sigma-Aldrich Chemical Co., and was used as received.
3.
Anhydrous methylene chloride was obtained by passage through a bed of activated alumina.
3
4.
Propanoyl chloride (98%) was purchased from Sigma-Aldrich Chemical Co., and was used as received.
5.
The reaction temperature was monitored internally with a thermocouple during addition of propanoyl chloride, such that the temperature never rose above +10 °C. The reaction was very vigorous and a thick suspension rapidly formed which was difficult to stir magnetically. The use of a mechanical stirrer is highly recommended for larger scale runs.
6.
The submitters monitored the progress of the reaction by
1H NMR spectroscopy. A small aliquot of the reaction mixture was removed by syringe and quenched with 1 mL of saturated aqueous NH
4Cl. The mixture was extracted with 3 mL of ethyl acetate. The organic extraction was dried over Na
2SO
4, filtered through glass wool, and concentrated by vacuum. The remaining material was dissolved in CDCl
3, and monitored by
1H NMR. Reaction completion was monitored by observance of product by
1H NMR analysis.
7.
The submitters used the 1-(dimethyl(phenyl)silyl)propan-1-one without purification for the next step. The amide is somewhat volatile and considerable amounts can be lost by extended exposure to high vacuum.
8.
The physical properties are as follows: bp 83–86 °C, (1.1 mmHg);
1H NMR
pdf (400 MHz, CDCl
3) δ 1.13 (t,
J = 7.4 Hz, 3 H), 2.32 (q,
J = 7.5 Hz, 2 H), 3.42–3.46 (t,
J = 4.9 Hz, 2 H), 3.58–3.62 (m, 2 H), 3.63–3.66 (m, 4 H).
13C NMR
pdf (101 MHz, CDCl
3) δ: 9.3, 26.2, 41.8, 45.7, 66.5, 66.9, 172.4. IR (film): 3493, 2977, 2920, 2854, 1644, 1463, 1435, 1380, 1362, 1300, 1274, 1233, 1116, 1069, 1035, 1008, 990, 942, 905 cm
−1; MS (EI, 70 eV)
m/z (rel. intensity) 144 (11), 143 [M
+, 98], 128 (131), 114 (21). Anal. Calcd for C
7H
13NO
2: C, 58.72; H, 9.15; N, 9.78. Found: C, 58.39; H, 9.32; N, 9.66. The submitters found their product to be > 99% pure by GC (Agilent 6890 Series, column: HP-5; 5% phenyl methyl siloxane, 30 m × 320 μm × 0.25 μm); T-program: 70 °C (1.0 min)→285 °C (25 °C/min); 6.03 min retention time) without distillation.
9.
The lithium wire (99.9% in mineral oil) was purchased from Sigma-Aldrich Chemical Co., and was pre-washed with pentanes (2 × 30 mL) to remove the oil and was charged in 1–3 mm segments. If larger (5–10 mm) segments were used the reaction took considerably longer time.
10.
Anhydrous tetrahydrofuran was obtained by passage through a bed of activated alumina.
3
11.
A moderate heat evolution was noted in the upper regions of the flask where the lithium floated.
12.
The reagent was unstable at room temperature and was kept in an ice bath (0 °C) when not stored in the freezer.
13.
Chlorodimethylphenylsilane (98%) was purchased from Gelest and was purified by distillation under vacuum through a short path distillation apparatus, topped with a thermometer, and attached to a receiving flask. (bp 75–77 °C (13–15 mmHg).
14.
The submitters monitored the progress of the reaction by TLC analysis on silica gel with 20% EtOAc-hexanes as eluent and visualization with potassium permanganate stain. The amide starting material had R
f = 0.14 and the acylsilane product had R
f = 0.62. Silyl impurities could be visualized at R
f = 0.36 and R
f = 0.72.
15.
The checkers found that chromatographic purification was necessary to remove the silicon-containing impurities (mostly disilane and disiloxane). However, chromatography with room temperature solvents led to a significant exotherm (and decomposition) when loading the acylsilane on silica gel (Silicycle Inc. Ultra Pure Silica Gel Silia
Flash® P60. Grade: 230–400 mesh (40–63 microns), 60 Å, catalog number R12030B, pH=6.9, water content 6.0%). The acylsilane had an R
f = 0.22 (hexane/CH
2Cl
2, 1/1, UV, I
2, CAN) and the major impurities had R
f = 0.43, 0.63, and 0.68.
16.
Analytical and spectral data are as follows:
1H NMR
pdf (500 MHz, CDCl
3) δ: 0.51 (s, 6 H), 0.92 (t,
J = 7.3 Hz, 3 H), 2.61 (q,
J = 7.2 Hz, 2 H), 7.36–7.44 (m, 3 H), 7.54–7.58 (m, 2 H).
13C NMR
pdf (125 MHz, CDCl
3 δ: −4.8, 6.1, 41.9, 128.1, 129.8, 133.9, 134.6, 246.2.
29Si NMR (60 MHz, CDCl
3) δ: −17.01. IR (film) 2972, 2937, 2898, 1644, 1429, 1403, 1373, 1250, 1111, 1032, 834 cm
−1. MS (EI, 70 eV)
m/z (rel. intensity) 191 (4), 163 (20), 137 (12), 136 (19), 135 (100), 105 (11). Anal. Calcd for C
11H
16OSi: C, 68.69; H, 8.39. Found: C, 68.52; H, 8.25.
17.
A second run on this scale provided 5.46 g (68%) of the acylsilane. The submitters obtained an 85% yield of material that was still contaminated with disilane and disiloxane.
Handling and Disposal of Hazardous Chemicals
The procedures in this article are intended for use only by persons with prior training in experimental organic chemistry. All hazardous materials should be handled using the standard procedures for work with chemicals described in references such as "Prudent Practices in the Laboratory" (The National Academies Press, Washington, D.C., 2011 www.nap.edu). All chemical waste should be disposed of in accordance with local regulations. For general guidelines for the management of chemical waste, see Chapter 8 of Prudent Practices.
These procedures must be conducted at one's own risk. Organic Syntheses, Inc., its Editors, and its Board of Directors do not warrant or guarantee the safety of individuals using these procedures and hereby disclaim any liability for any injuries or damages claimed to have resulted from or related in any way to the procedures herein.
3. Discussion
In the presence of a strong nucleophile, acylsilanes have the ability undergo a Brook rearrangement (1,2-silyl shift from carbon to oxygen), which has a firmly established synthetic utility.
4 This tactic has been used in various umpolung approaches to carbonyl anions
5 by generating nucleophilicity on the normally electrophilic carbonyl carbon. To exploit this reactivity, acylsilanes have been employed in the synthesis of enolsilanes
6 and alcohols
7 in addition to providing direct access to homoenolates upon addition of vinyl or alkynyl organometallic reagents.
8 More recently, acylsilanes that undergo the Brook rearrangement have been utilized in tandem annulation reactions,
9 and new catalytic acyl anion addition reactions have been developed taking advantage of the unique benefits of the resulting acyl anions.
10 More specifically, acylsilanes in our laboratory have been employed as acyl anion equivalents in the presence of catalytic quantities of thiazolium salts (20–30 mol%) to carry out sila-Stetter reactions,
11 tandem one-pot sila-Stetter/Paal-Knoor transformations,
12 and the direct syntheses of α-amino ketones.
13 However, although acylsilanes are useful entities, standard procedures for accessing these compounds have numerous limitations such as low overall efficiency and a reliance on stoichiometric quantities of a transition metal.
There are many synthetic approaches to construct the carbonyl carbon-silicon bond.
14 Unconventional approaches include the conversion of α,α-dibromobenzylsilanes to acylsilanes on silica gel
15 and the hydroboration of silylalkynes.
16 The trapping of deprotonated dithianes with chlorosilanes has been employed for many years, but this strategy always requires the unmasking of the α-silyl dithiane, which is not always compatible with the substrate.
17 Additionally, benzotriazol-1-yl phenoxyalkanes are useful precursors to a variety of acylsilane structures.
18 Not surprisingly, many of these processes are suboptimal because of poor availability of starting materials or their lack of atom economy.
19 α,β-Unsaturated acylsilanes can be prepared either by a Horner-Wadsworth-Emmons reaction of α-(phosphonoacyl)-silanes
20 or the silyl-Wittig rearrangement of allylic alcohols followed by oxidation.
21 Interestingly, the palladium-catalyzed conversion of aryl acid chlorides to aryl acylsilanes has been reported, but this reaction is limited to the trimethylsilyl group and undergoes decarbonylation with electron deficient aromatic systems.
22
The addition of anionic silyl nucleophiles to acid chlorides is typically the most direct method for the synthesis of acylsilanes, but this method requires at least two equivalents of the silyllithium reagent and suffers largely from the need for a stoichiometric amount of copper(I) cyanide required for the reaction to proceed in high yield.
23 Unfortunately on a preparative scale, this process becomes prohibitive. As an alternate approach, Fleming and coworkers first noted that the addition of silyl anions to amides afforded acylsilanes, but this process was not adequately explored as a method to construct acylsilanes.
24
Because the direct addition of organometallic reagents to morpholine amides without over-addition is possible,
25 and more economical than the corresponding Weinreb amides, a direct and efficient synthesis of acylsilanes from amides could be developed.
26 The use of the morpholine amide minimizes over-addition of the silyl nucleophile and also provides the highest yields of the amides surveyed.
The extension of this method to the use of aromatic morpholine amides does not yield the desired acylsilanes. Unlike with the alkyl morpholine amides, additions of silyl anions to aromatic amides usually results in a Brook rearrangement, presumably due to stabilization of the resulting carbanion by the aromatic moiety. Surprisingly, this anion subsequently undergoes an α-elimination of the silyloxy substituent and the ensuing carbene either encounters an additional equivalent of the silyllithium species or dimerizes.
27
The overall process to prepare alkyl substituted acylsilanes from morpholine amides demonstrated in this procedure has been optimized for a preparative scale, avoids the use of transition metals, and tolerates varied alkyl amide structures, including various protecting groups.
Appendix
Chemical Abstracts Nomenclature (Collective Index Number);
(Registry Number)
1-Morpholinopropan-1-one:
4-(1-oxopropyl)-morpholine; (30668-14-5)
Morpholine; (110-91-8)
Propanoyl chloride; (79-03-8)
Chlorodimethylphenylsilane; (768-33-2)
1-(Dimethyl(phenyl)silyl)propan-1-one:
Dimethyl(1-oxopropyl)phenylsilane; (128084-44-6)
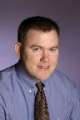 |
Karl A. Scheidt was born in 1972. He studied chemistry at the University of Notre Dame in the laboratories of Marvin J. Miller. His graduate studies were conducted at Indiana University and the University of Michigan under the direction of William R. Roush. Following his graduate research, he was a NIH Postdoctoral Fellow with David Evans at Harvard University. Since starting his independent career at Northwestern in 2002, his research has focused on the development of new catalytic methods and their applications in organic synthesis. He is the recipient of an Amgen Young Investigator Award, an Abbott Laboratories Young Investigator Award, the Boehringer-Ingelheim New Investigator Award in Organic Chemistry, and a Northwestern University Distinguished Teaching Award. |
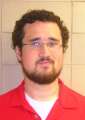 |
Robert B. Lettan II was born in Rochester, NY in 1979. He then matriculated at Otterbein College in Columbus, OH, where he received his B. S. in chemistry in 2002. In the fall of 2002, Robert began his doctoral studies at Northwestern University and joined the research group of Professor Karl Scheidt. At Northwestern he has conducted research involving the Lewis base activation of silylalkynes and novel synthetic methods using acylsilanes. In 2006, he was awarded the distinguished Edmund W. Gelewitz award for research and service associated with the Northwestern University Chemistry Department and the surrounding community. |
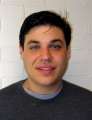 |
Benjamin C. Milgram was born in Chicago, IL in 1985. He started his undergraduate studies at Northwestern University in 2003. He joined the research group of Karl Scheidt in 2004. During his time in the group he has been awarded a Northwestern University undergraduate fellowship (2004) for work on the synthesis of acylsilanes, a Sigma-Aldrich Research Fellowship (2005) for work on thiazolium catalyzed conjugate additions, and the Katherine Kriegbaum Scholarship for work on the synthesis of isoflavone derivatives (2006). |
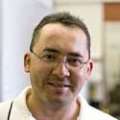 |
Ramil Y. Baiazitov graduated with a Diploma in Chemistry from Moscow State University in 1993. As an undergraduate student, he worked under supervision of Prof. Eduard A. Karakhanov on hydroformylation of olefins. After working at a petrochemical company in Ufa, Russia and serving in the Russian Army for almost two years, he worked for three years at the University of Minnesota, Duluth (with Dr. Pavel A. Krasutsky). In Prof. Denmark's group at the University of Illinois, his Ph.D. research is focused on the tandem double intramolecular variant of the [4+2]/[3+2] nitro olefin cycloaddition and its application to total synthesis. |
Copyright © 1921-, Organic Syntheses, Inc. All Rights Reserved