Org. Synth. 2007, 84, 317
DOI: 10.15227/orgsyn.084.0317
1-(4-ACETYLPHENYL)-2-PHENYLETHANE from POTASSIUM 2-PHENETHYLTRIFLUOROBORATE and 4-BROMOACETOPHENONE
Submitted by Gary A. Molander and Daniel E. Petrillo
1.
Checked by Scott E. Denmark and Christophe Eggertswyler.
1. Procedure
A. Preparation of Potassium 2-Phenethyltrifluoroborate. A single-necked, 500-mL round-bottomed flask, equipped with a magnetic stirring bar, a 125-mL pressure-equalizing addition funnel, and connected by a three-way stopcock fitted with a rubber septum to a vacuum-argon manifold, is flame-dried under vacuum (0.3 mm Hg), then is flushed with dry argon. THF (90 mL) is added via syringe to the addition funnel, followed by trimethyl borate (10.0 mL, 89.2 mmol, 1.5 equiv) (Note 1). After the addition of the trimethyl borate to the round-bottomed flask, additional THF (30 mL) (Note 1) is added via syringe to rinse the addition funnel. The solution is then cooled in a dry ice/isopropyl alcohol bath. Phenethylmagnesium chloride (60.0 mL of a 1.0 M solution in THF, 60.0 mmol) (Note 2) is then added dropwise over 1 h via the addition funnel. The mixture is stirred in the dry ice/isopropyl alcohol bath for 1.5 h, then is allowed to warm to room temperature over 1 h. The resulting white slurry is then cooled in an ice/water bath and methanol (60 mL) (Note 3) is charged into the addition funnel by syringe. The methanol is added over 15 min to dilute the slurry (Note 4). The argon inlet is removed and a solution of potassium hydrogen fluoride (80.0 mL of 4.5 M aqueous solution, 360 mmol, 6.0 equiv) (Note 5) is then charged into the addition funnel and is added dropwise over 15 min. The resulting solution is stirred for 1 h in the ice/water bath, and then is concentrated on a rotary evaporator (60 °C water bath, 9 mm Hg). The resulting white solids are placed on a high-vacuum line (0.3 mm Hg) for 36 h until the solids appear dry (Note 6). Acetone (120 mL) (Note 7) is added and the solids are broken up into a fine powder with a spatula. The suspension is then placed on a rotary evaporator and heated (40 °C) with rotation under atmospheric pressure for 5–10 min and filtered over a 14 g pad of Celite in a glass-fritted filter funnel. Acetone (120 mL) is added to the solid remaining in the flask, the suspension is placed on a rotary evaporator and heated (40 °C) with rotation under atmospheric pressure for 5–10 min and filtered over the same Celite pad. This process is repeated one additional time (Note 8). The combined filtrates are concentrated on a rotary evaporator (60 °C water bath) to a white solid, which is dissolved in a minimal amount of dry acetone (~25 mL). Diethyl ether (30 mL) is then added to precipitate the product. The white slurry is allowed to cool first to 23 °C, and then is cooled in an ice/water bath for 15 min. The slurry is placed in a refrigerator (4 °C) for 1 h, and is then filtered to give 9.84 g (77%) of a white solid (Note 9).
B. Preparation of 1-(4-Acetylphenyl)-2-phenylethane. A three-necked, 250-mL, round-bottomed flask, equipped with a reflux condenser, a rubber septum, a glass stopper, a magnetic stirring bar, and connected to a vacuum-argon manifold, is charged sequentially with potassium 2-phenethyltrifluoroborate (7.77 g, 36.6 mmol, 1.05 equiv), 4-bromoacetophenone (6.97 g, 35.0 mmol), potassium carbonate (14.5 g, 105 mmol, 3.0 equiv), triphenylphosphine (0.275 g, 1.05 mmol, 0.03 equiv) and palladium acetate (0.118 g, 0.53 mmol, 0.015 equiv) (Note 10). The flask is evacuated and filled with dry argon three times. Toluene (105 mL) (Note 11) and H2O (21 mL) are added, and the resulting two-phase mixture is stirred in a 95 °C oil bath until consumption of the 4-bromoacetophenone is indicated by GC analysis (Note 12). The mixture is cooled to room temperature and then is transferred to a 250-mL separatory funnel. The flask is rinsed with toluene (20 mL) and H2O (20 mL). The layers are shaken and separated. The organic layer is washed with 10% aq. citric acid solution (20 mL) (Note 13) and saturated aq. NaCl solution (20 mL). The organic layer is dried over anhydrous Na2SO4 (0.780 g) and filtered through a medium porosity glass-fritted funnel, rinsing the drying agent with toluene (20 mL). The resulting solution is concentrated (50 °C water bath, 9 mmHg) to a light yellow solid (Note 14), which is crystallized from methanol to give 5.79 g (74%, two crops) (Notes 15, 16) of a light tan solid. Recrystallization of 0.3 g of this solid from hot methanol (2 mL) provided white, crystalline material (0.25 g) of analytical purity (Note 17).
2. Notes
1.
Trimethyl borate (98%) was obtained from Aldrich Chemical Company and was distilled from sodium. THF (Fischer, HPLC Grade) was dried by percolation through a column packed with neutral alumina and a column packed with Q5 (a supported copper catalyst for removing oxygen) under a positive pressure of argon.
2.
Phenethylmagnesium chloride (1.0 M in THF) was obtained from Aldrich Chemical Company and was used as received.
3.
Methanol (ACS Grade) was obtained from Aldrich Chemical Company and was distilled continuously from Mg(OMe)
2.
4.
At this stage the solution may be transferred to a Nalgene vessel to avoid etching of the glass flask by the KHF
2.
5.
Potassium hydrogen fluoride (p.a. >99%) was obtained from Fluka and was used as received.
6.
Care was taken to ensure that the solids are free of water or else inorganic fluoride contaminated the final product.
7.
Acetone (Spectroscopic Grade) was obtained from Aldrich Chemical Company and was used as received.
Diethyl ether (ACS Grade) was obtained from Fischer Scientific and was used as received.
8.
The desired product was soluble in acetone whereas the inorganic salts were not. This extractive process ensured that any product trapped within the inorganic solids would be dissolved.
9.
Analytical data: mp: 332–335 °C (sealed tube). IR cm
−1: 3026, 2921, 2847;
1H NMR
pdf (600 MHz, acetone-
d6) δ: 0.51 (br, 2 H), 2.56 (dt,
J = 8.8, 4.7 Hz, 2 H), 7.00–7.05 (m, 1 H), 7.14–7.17 (m, 4 H);
13C NMR
pdf (150 MHz, acetone-
d6) δ: 32.1, 124.5, 127.9, 128.0, 148.5;
11B NMR
pdf (192.4 MHz, acetone-
d6) δ 5.48;
19F NMR
pdf (470 MHz, acetone-
d6) δ −142.32; MS (ES):
m/z 173.1 (100) Anal. Calcd for C
9H
9BF
3K: C, 45.31; H, 4.28; F, 26.88. Found: C, 45.03; H, 4.29; F, 25.38.
10.
4-Bromoacetophenone (98%) was obtained from Aldrich Chemical Company and was used as received.
Potassium carbonate (ACS Grade) was purchased from Fisher Scientific and was used as received.
Triphenylphosphine (ReagentPlus Grade, 99%) was obtained from Aldrich Chemical Company and was used as received.
Palladium acetate was obtained from Frontier Scientific and was used as received.
11.
Toluene (ACS Grade, 99.5+%) was obtained from Aldrich Chemical Company and was used as received.
12.
The reaction was assayed as follows: an aliquot (20 μL) was removed and filtered through a small pad of silica gel in a Pasteur pipette, rinsing with
EtOAc (1.5 mL). The sample was then injected on an HP 5890 GC with an HP 1 column (100 °C for 1 min., ramp to 250 °C at 25 °C/min.). Typical retention times were: 4-bromoacetophenone: 4.1 min, 1-(4-acetylphenyl)-2-phenylethane: 7.4 min. The reaction generally required 16–20 h.
13.
Citric acid monohydrate (Baker Analyzed Reagent) was obtained from J. T. Baker Chemical and was used as received.
14.
The submitters found that the crude product was a dark yellow solid that needed to be decolorized with activated carbon in MeOH prior to recrystallization.
15.
The crude product was slurried in
MeOH (25 mL) in a
100-mL flask and heated to 50 °C in an oil bath. After dissolution, the solution was allowed to cool to room temperature. It was then placed in an ice/water bath for 1 h, after which crystals were removed by filtration. The filtrate was concentrated (50 °C water bath) to ~ 10 mL and cooled to 0 °C, after which a second crop of the crystals was collected via filtration. This material had a melting point of 66–68 °C.
16.
The submitters noted that the product may also be purified by silica gel column chromatography (10% EtOAc/hexanes as eluent, R
f = 0.34) to give an 89% yield of product of unspecified purity.
17.
Analytical data: mp: 68–72 °C (lit. mp: 68–70 °C).
2 IR cm
−1: 1676, 1601;
1H NMR
pdf (600 MHz, CDCl
3) δ: 2.58 (s, 3 H), 2.92–3.01, (m, 4 H), 7.15–7.30 (m, 7 H), 7.88 (d,
J = 8.3 Hz, 2 H);
13C NMR
pdf (150 MHz, CDCl
3) δ: 26.5, 37.3, 37.8, 126.1, 128.3, 128.4, 128.5, 128.7, 135.1, 141.0, 147.4, 197.7. MS (EI, 70 eV):
m/z 224 (M
+, 44), 209 (12), 181 (8), 133 (12), 105 (8), 91 (100), 65 (7); Anal. Calcd for C
16H
16O: C, 85.68; H, 7.19. Found: C, 85.59; H, 7.31.
Handling and Disposal of Hazardous Chemicals
The procedures in this article are intended for use only by persons with prior training in experimental organic chemistry. All hazardous materials should be handled using the standard procedures for work with chemicals described in references such as "Prudent Practices in the Laboratory" (The National Academies Press, Washington, D.C., 2011 www.nap.edu). All chemical waste should be disposed of in accordance with local regulations. For general guidelines for the management of chemical waste, see Chapter 8 of Prudent Practices.
These procedures must be conducted at one's own risk. Organic Syntheses, Inc., its Editors, and its Board of Directors do not warrant or guarantee the safety of individuals using these procedures and hereby disclaim any liability for any injuries or damages claimed to have resulted from or related in any way to the procedures herein.
3. Discussion
The metal-catalyzed cross-coupling reaction between organometallic reagents with electrophiles has emerged as one of the most powerful methods for carbon-carbon bond formation.
3 The Suzuki-Miyaura
4 reaction using organoboron reagents is among the most important, due its tolerance of a broad range of functional groups as well as its formation of non-toxic byproducts. The use of alkylboron reagents has received special attention because of their utility in natural product synthesis.
5
The coupling of alkylboron reagents has generally been performed with trialkylboranes (R
3B), alkylborinate esters (R
2BOR'), alkylboronic acids [RB(OH)
2] or boronate esters [RB(OR')
2]. Trialkylboranes such as 9-BBN
6 derivatives are among the most common, although they present problems such as functional group intolerance, air sensitivity, and a lack of atom economy. The use of alkylboronate esters often results in low yields in coupling reactions unless highly toxic thallium bases are used.
7 Additionally, these species are often hydrolytically unstable. The coupling of alkylboronic acids
8 has shown more promise, although their tendency to form cyclic anhydrides (boroxines) often makes determining their precise stoichiometry difficult.
Recently, potassium organotrifluoroborates (RBF
3K) have been shown to be highly effective coupling partners in the Suzuki-Miyaura reaction.
9 They are easily prepared on large-scale (>200 g) by the addition of KHF
2 (an inexpensive fluoride source) to various organoboron reagents such as boronic acids, boronate esters, and organodihaloboranes. Their advantages over traditional organoboron reagents include their greater nucleophilicity, air stability, and atom economy. Initial reports described their use in the palladium-catalyzed coupling with arenediazonium
10 or diaryliodonium
11 salts. Previous reports from our laboratory have shown the successful cross-coupling of potassium alkyl-,
12 alkenyl-,
13 aryl-,
14 and alkynyltrifluoroborates
15 with aryl- and alkenyl halides as well as triflates using catalytic PdCl
2(dppf)•CH
2Cl
2 and Cs
2CO
3 or Et
3N as a base. In the case of aryltrifluoroborates, it was also shown that ligandless conditions using Pd(OAc)
2 and K
2CO
3 can be used.
16 Batey and Quach have reported similar cross-coupling reactions using tetraalkylammonium trifluoroborates.
17 Recently, functionalized organotrifluoroborates such as epoxidized
18 and dihydroxylated
19 potassium alkyltrifluoroborates have been coupled to electrophiles under palladium catalysis. The procedure described above represents a typical coupling reaction in which a commercially available, easily-handled potassium alkyltrifluoroborate is coupled to a common aryl electrophile.
Appendix
Chemical Abstracts Nomenclature (Collective Index Number);
(Registry Number)
Trimethyl borate; (121-43-7)
Phenethylmagnesium chloride:
Magnesium, chloro(2-phenylethyl)-; (90878-19-6)
Potassium hydrogen fluoride: (7789-29-9)
Potassium 2-phenethyltrifluoroborate:
Borate(1-), trifluoro(2-phenylethyl)-, potassium, (T-4)-: (329976-74-1)
4-Bromoacetophenone:
Ethanone, 1-(4-bromophenyl)-: (99-90-1)
Triphenylphosphine; (603-35-0)
Palladium acetate; (3375-31-3)
1-(4-Acetylphenyl)-2-phenylethane:
1-[4-(2-Phenylethyl)phenyl]-ethanone; (785-78-4)
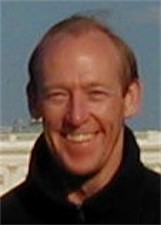 |
Professor Gary Molander received his B.S. degree at Iowa State University in 1975, working with Professor Richard C. Larock. He obtained his Ph.D. degree in 1979 under the direction of Professor Herbert C. Brown. He joined Professor Barry Trost's group at the University of Wisconsin, Madison as a National Institutes of Health postdoctoral fellow in 1980, and in 1981 he accepted an appointment at the University of Colorado, Boulder. In 1999 he joined the faculty at the University of Pennsylvania. His research interests focus on the development of new synthetic methods for organic synthesis and natural product synthesis.
|
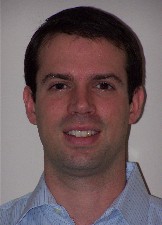 |
Daniel E. Petrillo received an A.B. in chemistry with honors from Princeton University in 1999. His senior thesis research was conducted in the laboratory of Professor Martin F. Semmelhack. From 1999 to 2002 he was employed as a chemist in the department of Process Research at Merck. He then entered graduate school at the University of Pennsylvania where his research has centered on synthetic methods using organotrifluoroborates under the guidance of Professor Gary A. Molander.
|
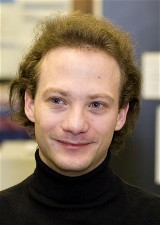 |
Christophe Eggertswyler was born and raised in Switzerland. He received his Diploma In Chemistry from the University of Fribourg, where he performed undergraduate research on the synthesis and ab initio computation of vibrational optical activity of sulfoxides. After graduation, he decided to stay in Fribourg where he joined the research group of Professor Titus A. Jenny. He investigated the synthetic potential of new iron tricarbonyl complexes in an organometallic approach to the taxane skeleton synthesis. After completion of his Ph.D. thesis in 2004, he joined the research group of Professor Scott E. Denmark at the University of Illinois at Urbana Champaign in 2005 as a postdoctoral research associate. His research focuses on the mechanistic study of the transmetallation event in cross-coupling reactions.
|
Copyright © 1921-, Organic Syntheses, Inc. All Rights Reserved