Org. Synth. 2007, 84, 347
DOI: 10.15227/orgsyn.084.0347
SYNTHESIS OF AN N-SUBSTITUTED LACTAM USING AN INTRAMOLECULAR SCHMIDT REACTION: FORMATION OF 2,3,11,11a-TETRAHYDRO-1H-BENZO[d]PYRROLO[1,2-a]AZEPIN-5(6H)-ONE
Submitted by Scott Grecian and Jeff Aubé.
Checked by Dalian Zhao and David J. Mathre.
1. Procedure
Caution! Sodium azide is toxic as well as heat shock sensitive. Metallicsharp surfaces should be avoided.
A. Methyl 2-oxo-1,2,3,4-tetrahydronaphthalene-1-carboxylate (1). An oven-dried 250-mL three-necked, round-bottomed flask equipped with a glass stopper, thermometer, rubber septum, large magnetic stirring bar, and two venting needles is charged with 8.0 g (200 mmol) of 60% sodium hydride in mineral oil by temporarily removing the glass stopper. The suspension is then washed with three 30 mL portions of anhydrous hexanes using a syringe through the septum. Anhydrous dimethyl carbonate is added (Note 1) (120 mL) and the mixture is cooled to 5 °C with an ice bath. β-Tetralone (Note 2) (13.3 mL, 100 mmol) is added via syringe over 20 min. The resulting green/brown mixture is then stirred for 1 h, at which time the ice bath is removed and the reaction is warmed to 23 °C. After 12 h, the glass stopper is removed and the reaction flask is fitted with a reflux condenser and heated to reflux for 1 h in an oil bath (Note 3). The brown mixture is cooled to room temperature, the condenser is removed, 20 mL of water is added dropwise and the mixture is poured into a 1-L separatory funnel followed by the addition of 200 g of ice and the slow addition of 250 mL of 1.0 M HCl. The mixture is extracted with diethyl ether (3 × 100 mL). The ethereal extracts are combined and washed once with 150 mL of brine and dried with magnesium sulfate, filtered and concentrated to give 20–25 g of a red oil. The oil is transferred to a 100-mL round-bottomed flask and topped with a short path distillation head fitted with a thermometer and four receiving flasks submerged in an ice bath. Vacuum distillation is performed at 0.9 mm Hg while the oil bath temperature is increased gradually to 185 °C. The first several drops are collected between 136 and 141 °C and discarded. Methyl 2-oxo-1,2,3,4-tetrahydronaphthalene-1-carboxylate (1) is collected between 138 and 141 °C as a pale yellow oil (16.9 – 17.8 g, 83–87%) (Notes 4 and 5).
B. 3-(3'-Chloropropyl)-3,4-dihydronaphthalen-2(1H)-one (2). To an oven-dried 250-mL, three-necked, round-bottomed flask equipped with a rubber septum, a thermometer, a glass stopper, and a magnetic stir bar is added 120 mL of freshly distilled THF and diisopropylamine (15.1 mL, 108 mmol) (Note 6) by syringe through the septum. After cooling the flask to 0 °C in an ice bath, 45 mL of n-butyllithium (105 mmol, 2.32 M /hexanes) are added dropwise over 10 min while maintaining the temperature at or below 10 °C (Note 7). After an additional 45 min, methyl 2-hydroxy-3,4-dihydronaphthalene-1-carboxylate (1) (10.0 g, 49 mmol) dissolved in 25 mL of THF is added via cannula through the syringe over 15 min while maintaining the reaction temperature below 10 °C (Note 8). After 15 min, the ice bath is removed and the reaction is stirred for 1 h at which time it is cooled to 0 °C in an ice bath. After an additional 30 min, 1-chloro-3-iodopropane (6.22 mL, 59 mmol) (Note 9) is added in several portions via syringe over 25 min while maintaining the reaction temperature below 10 °C. The ice bath is removed after 30 min, and the reaction is stirred for an additional h, at which time the reaction is quenched by the slow addition of 50 g of ice. The mixture is transferred to a separatory funnel and 150 mL of 1.0 M HCl is added slowly. The organic layer is separated and the mixture is extracted with diethyl ether (2 × 100 mL). The combined organic layers are washed once with 175 mL of brine, dried with magnesium sulfate, filtered and concentrated to give a red oil (15.2–15.8 g) (Note 10). The oil is transferred to a 100-mL, single-necked, round-bottomed flask equipped with a stir bar and a reflux condenser, and the oil is dissolved in 35 mL of glacial acetic acid. Concentrated HCl (5 mL) is added. The mixture is stirred at room temperature for 30 min, then placed in an oil bath and heated to 110 °C for 3 h, then cooled to room temperature and poured into a separatory funnel followed by the addition of 100 mL of water and 75 mL of diethyl ether. The aqueous layer is extracted with diethyl ether (2 × 75 mL). The combined extracts are washed with a saturated solution of sodium bicarbonate (3 × 100 mL) (Note 11). The organic layer is then washed once with 150 mL of brine, dried over magnesium sulfate, filtered and concentrated to a red oil (11.7–12.0 g). The oil is chromatographed over 100 g of silica gel (25 × 5 cm) starting with 500 mL of 5% ethyl acetate/hexanes followed by 500 mL of 10% ethyl acetate/hexanes. The desired product is collected in fractions 28 to 40 (25 mL fractions), which upon concentration, gives 8.1–8.2 g (73–74% from 1) of 3-(3'-chloropropyl)-3,4-dihydronaphthalen-2(1H)-one (2) as a light yellow oil (Note 12).
C. 3-(3'-Azidopropyl)-3,4-dihydronaphthalen-2(1H)-one (3). In a 100-mL single-necked round-bottomed flask equipped with a stir bar is dissolved 3-(3-chloropropyl)-3,4-dihydronaphthalen-2(1H)-one (2) (5.0 g, 22 mmol) in 60 mL of anhydrous N,N-dimethylformamide. A blasting shield is placed around the reaction flask and sodium iodide (3.96 g, 26.4 mmol) (Note 13) and sodium azide (2.86 g, 44.0 mmol) (Note 14) are added. The mixture is stirred while immersed in an oil bath heated to 60 °C for 12 h, after which time the reaction mixture is poured into 150 mL of water and extracted with diethyl ether (2 × 100 mL) (Notes 15 and 16). The organic layer is washed with brine (3 × 150 mL), dried with magnesium sulfate, filtered and concentrated at or below 30 °C to a red oil (4.9–5.2 g). The oil is loaded on a column of 60 g of silica gel (18 × 5 cm) and eluted with 750 mL of 10% ethyl acetate/hexanes. The desired product is collected in fractions 18–28 (25 mL fractions) which, after concentration, gives 4.10–4.24 g (81–84%) of 3-(3'-azidopropyl)-3,4-dihydronaphthalen-2(1H)-one (3) as a red oil (Note 17).
D. 2,3,11,11a-Tetrahydro-1H-benzo[d]pyrrolo[1,2-a]azepin-5(6H)-one (4). An oven-dried, three-necked 100-mL round-bottomed flask is equipped with a thermometer, rubber septum, an elbow adapter connected to a tygon tube with an in-line bubbler, and a stir bar. 3-(3'-Azidopropyl)-3,4-dihydronaphthalen-2(1H)-one (4.0 g, 17.44 mmol) of (3) is added to the flask by temporarily removing the septum and cooled to 0 °C in an ice-bath. Trifluoroacetic acid (Note 18) (20 mL) is added dropwise through the septum by syringe over 15 min (Note 19). After 30 min, the ice bath is removed and the reaction is allowed to warms to room temperature. After an additional 30 min, 50 mL of water is added to the reaction, which is then poured into a separatory funnel. The aqueous layer is extracted with chloroform (3 × 40 mL). The organic extracts are combined and washed with 100 mL of a saturated solution of sodium bicarbonate, dried with magnesium sulfate, filtered and concentrated to an orange solid (3.7–4.0 g). The solid is dissolved in 5 mL of hot chloroform, loaded onto a column of 40 g of silica gel (10 × 5 cm) and eluted with 350 mL of 20% ethyl acetate/hexanes followed by 500 mL of ethyl acetate. The desired product is collected in fractions 19–34 (25 mL fractions) which, after concentration, gives 2.87–3.09 g (82–88%) of 2,3,11,11a-tetrahydro-1H-benzo[d]-pyrrolo[1,2-a]azepin-5(6H)-one (4) as an off-white solid (Note 20).
2. Notes
1.
Sodium hydride and dimethyl carbonate were purchased from Aldrich Chemical Company, Inc.
2.
3,4-Dihydronaphthalen-2(1H)-one (β-tetralone) was purchased from Aldrich Chemical Company, Inc.
3.
The reaction progress was monitored by silica gel thin layer chromatography.
4.
This compound was prepared according to the procedure described in Oommen, P. K.
Aust. J. Chem.
1976,
29, 1393-–1396.
5.
The product exhibited the following properties: R
f = 0.48 (1:9, ethyl acetate/hexanes); IR (neat): 1640, 1595, 1225 cm-1;
1H NMR
pdf (400 MHz, CDCl
3) δ: 2.59 (t,
J = 7.3 Hz, 2 H), 2.86 (t,
J = 7.3 Hz, 2 H), 3.96 (s, 3 H), 7.18–7.12 (m, 2 H), 7.25 (t,
J = 10.2 Hz, 1 H), 7.75 (d,
J = 6.7 Hz, 1 H), 13.39 (s, 1 H);
13C NMR (100 MHz, CDCl
3) δ: 27.8, 29.6, 51.8, 99.9, 125.0, 125.9, 126.5, 127.3, 131.4, 133.2, 172.5, 178.5; MS (FAB)
m/z (relative intensity): 204 (M+, 99), 172 (100); HRMS calcd for C
12H
13O
3 (MH+): 205.0865, found: 205.0853.
6.
Anhydrous
diisopropylamine was purchased from the Aldrich Chemical Company, Inc., and used without further purification.
7.
n-Butyllithium was purchased from the Aldrich Chemical Company, Inc., and titrated prior to use. For the titration procedure used, see: Kofron, W. G.; Baclawski, L. M.
J. Org. Chem. 1976,
41, 1879-–1880.
8.
A voluminous light yellow precipitate began to form near the end of the addition.
9.
1-Chloro-3-iodopropane was purchased from Aldrich Chemical Company, Inc.
10.
An aliquot (285 mg) was loaded on 10 g of silica gel and eluted with 5% ethyl acetate/hexanes. Concentration of the fractions containing the product gave 178 mg of methyl 3-(3-chloropropyl)-2-oxo-1,2,3,4-tetrahydronaphthalene-1-carboxylate as a light yellow oil. This product exhibited the following physical properties: R
f = 0.40 (1:9, ethyl acetate/hexanes); IR (neat): 3440, 1735, 1640, 1595 cm-1;
1H NMR
pdf (400 MHz, CDCl
3) δ: 1.51–1.57 (m, 1 H), 1.67–1.71 (m, 1 H), 1.84–1.93 (m, 2 H), 2.55–2.59 (m, 1 H), 2.66 (dd,
J = 4.9, 15.1 Hz, 1 H), 3.06 (dd,
J = 5.6, 15.1 Hz, 1 H), 3.53 (sextet,
J = 6.9 Hz, 2 H), 3.95 (s, 3 H), 7.09–7.16 (m, 2 H), 7.22 (dt,
J = 1.5, 7.1 Hz, 1 H), 7.73 (d,
J = 7.8 Hz, 1 H), 13.49 (s, 1 H);
13C NMR (100 MHz, CDCl
3) δ: 27.0, 30.3, 33.2, 38.5, 44.7, 51.9, 99.3, 125.2, 125.8, 126.5, 128.0, 130.6, 131.5, 172.7, 180.4; MS (FAB)
m/z (relative intensity): 280 (M+, 100), 248 (77); HRMS calcd for C
15H
17ClO
3 (M+): 280.0866, found: 280.0873.
11.
Significant gas evolution was observed upon addition of a saturated solution of sodium bicarbonate.
12.
The product exhibited the following properties: R
f = 0.41 (1:4, ethyl acetate/hexanes); IR (neat): 2955, 1710, 750 cm-1;
1H NMR (500 MHz, CDCl
3) δ: 1.58–1.62 (m, 1 H), 1.87–1.97 (m, 3 H), 2.48 (sextet,
J = 6.4 Hz, 1 H), 2.89 (dd,
J =10.3 Hz, 15.4, 1 H), 3.13 (dd,
J = 5.5 Hz, 15.4, 1 H), 3.58 (sextet,
J = 4.4 Hz, 2 H), 3.62 (d,
J = 8.1 Hz, 2 H), 7.14 (m, 1 H), 7.24 (m, 3 H);
13C NMR (125 MHz, CDCl
3) δ: 27.1, 30.1, 34.4, 44.4, 44.8, 46.7, 126.8, 126.9, 127.8, 128.0, 133.1, 135.6, 210.9; MS (FAB)
m/z (relative intensity): 223 (MH+, 100); HRMS calcd for C
13H
16ClO (MH+): 223.0890, found: 223.0890.
13.
Sodium iodide and sodium azide were purchased from the Aldrich Chemical Company Inc.
14.
Caution:
Sodium azide is explosive and toxic. Metallic and sharp surfaces should be avoided.
15.
Although the submitters have not experienced any explosions of alkyl azides, the substances should be treated with extreme caution.
16.
The reaction was monitored by silica gel thin layer chromatography.
17.
The product exhibited the following properties: R
f = 0.39 (1:4, ethyl acetate/hexanes); IR (neat): 2920, 2080, 1700 cm-1;
1H NMR
pdf (300 MHz, CDCl
3) δ: 1.49 (ddd,
J = 6.8, 9.2, 13.3 Hz, 1 H), 1.69 (ddd,
J = 6.8, 6.8, 14.0 Hz, 1 H), 1.79–1.93 (m, 1 H), 2.46 (sextet,
J = 6.4 Hz, 1 H), 2.85 (dd,
J = 10.3, 15.4 Hz, 1 H), 3.11 (dd,
J = 5.6, 15.5 Hz, 1 H), 7.15–7.23 (m, 3 H), 3.29 (dt,
J = 1.4, 6.8 Hz, 2 H), 3.60 (d,
J = 6.4 Hz, 2 H) 7.05–7.15 (m, 1 H);
13C NMR (100 MHz, CDCl
3) δ: 26.5, 27.0, 34.5, 44.5, 47.0, 51.4, 126.9, 127.0, 127.9, 128.1, 133.2, 135.7, 211.0; Anal calcd for C
13H
15N
3O: C, 68.10; H, 6.59; N, 18.33; found C, 68.38; H, 6.71; N, 17.98.
18.
Trifluoroacetic acid was purchased from the Aldrich Chemical Company, Inc.
19.
The reaction turned purple, significant gas evolution was observed and heat was evolved during the addition of trifluoroacetic acid.
20.
The product exhibited the following properties: mp 120–121 °C; R
f = 0.17 (2:3, ethyl acetate/hexanes); IR (neat): 2955, 1625 cm-1;
1H NMR
pdf (300 MHz, CDCl
3) δ: 1.69–2.25 (m, 3 H), 2.18–2.31 (m, 1 H), 2.95–3.14 (m, 2 H), 3.47–3.66 (m, 3 H), 4.08–4.22 (m, 2 H), 7.02–7.22 (m, 4 H);
13C NMR (100 MHz, CDCl
3) δ: 23.0, 33.7, 39.4, 44.1, 46.7, 57.1, 127.0, 127.5, 130.3, 131.0, 132.5, 136.5, 170.0; MS (EI)
m/z (relative intensity): 201 (M+, 100); HRMS calcd for C
13H
15NO (M+): 201.1154, found: 201.1144. Anal. Calcd for C
13H
15NO: C, 77.58; H, 7.51; N, 6.96. Found: C, 77.55; H, 7.38; N, 7.12.
Handling and Disposal of Hazardous Chemicals
The procedures in this article are intended for use only by persons with prior training in experimental organic chemistry. All hazardous materials should be handled using the standard procedures for work with chemicals described in references such as "Prudent Practices in the Laboratory" (The National Academies Press, Washington, D.C., 2011 www.nap.edu). All chemical waste should be disposed of in accordance with local regulations. For general guidelines for the management of chemical waste, see Chapter 8 of Prudent Practices.
These procedures must be conducted at one's own risk. Organic Syntheses, Inc., its Editors, and its Board of Directors do not warrant or guarantee the safety of individuals using these procedures and hereby disclaim any liability for any injuries or damages claimed to have resulted from or related in any way to the procedures herein.
3. Discussion
Although the Schmidt reaction of hydrazoic acid with ketones has been known for many years, the corresponding reaction of ketones with alkyl azides, affording
N-substituted lactams, is of much more recent vintage.
1–4 In the 1940s, several researchers reported that the reaction of methyl azide with simple ketones using standard Schmidt reaction conditions (concentrated H
2SO
4) did not lead to any lactam products.
5,6 In the following decade, Boyer found that amides could be formed in modest yields (≥30%) when aromatic aldehydes were allowed to react with
n-hexyl azide under H
2SO
4 promotion, but reported that these conditions failed when applied to ketones.
7 In the early 1990s, it was learned that Schmidt reactions of alkyl azides and ketones could be actuated by carrying out intramolecular reactions in δ- or ε-azido ketones.
8
The intramolecular Schmidt reaction requires that the ketone and azide group be separated by four or five atoms (usually carbons), with the former being significantly more favorable.
8,9 Thus, Schmidt reactions of δ-azido ketones can be promoted by strong protic acids, as exemplified in the present procedure, while the homologous substrates require stronger Lewis acid promotion and are generally much more sluggish (Table 1). This is presumably because the former reactions proceed through a six-membered azidohydrin intermediate en route to products, whereas the seven-membered ring version of this intermediate is less favored (Scheme 1). In a specialized example, it was shown that advantage could be taken of the dependence of reactivity on tether length to effect a regioselective reaction in a complex example of the reaction on a diketone reactant.
10 Subsequently, it was found that the more stringent conditions used to carry out Schmidt reactions of ε-azido ketones could also be applied to intermolecular reactions of simple alkyl azides and ketones, although this reaction is of severely limited scope and frequently plagued by complicating (if sometimes interesting) side reactions.
11,12 Conversely, the intramolecular Schmidt reaction is very forgiving with respect to substitution pattern and to the nature of the reacting ketone, with successful reactions being observed to date in ketones containing from 3 to 12 members. Aldehydes also react, but can give rise to either
N-substituted or -unsubstituted lactams depending on whether an alkyl group or a hydrogen atom migrates.
Some other features of the intramolecular Schmidt reaction are that it is highly regioselective and, where relevant, stereoselective. Thus, the product formed in a typical example of this reaction is almost always the fused lactam formed by migration of the α-carbon between the carbonyl and azide. However, a few examples of bridged adducts have been observed in specialized substrates.
10,13,14 As is common for related processes like the classical Schmidt reaction and the Baeyer-Villiger reaction, the intramolecular Schmidt reaction is stereoselective insofar as the α-carbon migrates with retention of configuration.
9 An important practical consideration, however, is that apparent loss of stereoselectivity can be observed due to the fact that the α-hydrogen is acidic and therefore this center is subject to enolization and epimerization. This is particularly problematic for sluggish subsnstrates that require heating or extended reaction times.
15
Scheme 1
Table 1. Intramolecular Schmidt Reactions of Azido Ketones
Over the same time period that saw the development of the intramolecular Schmidt reaction, a number of related processes were also reported. These included the reactions of alkyl azides with carbocations generated from alkenes
16–19 or other functional groups,
20,21 principally developed by Pearson but explored by other researchers as well. Subsequent to the discovery of the intramolecular Schmidt reaction, this laboratory has also explored the related reactions of 2- or 3-hydroxyalkyl azides with ketones, some of which can be used to effect an asymmetric nitrogen insertion reaction.
22–25 These, along with other important developments in modern alkyl azide chemistry, have been summarized in an exhaustive review article.
26
The main utility of the present reaction is in the preparation of multicyclic nitrogen containing rings in complex alkaloid synthesis. Some targets that have been explored using this chemistry include homoerythrina alkaloids,
27 indolizidines isolated from South American frogs,
28,29 gephyrotoxin,
30 sparteine,
31 stenine,
13 and aspidospermidine.
10
Appendix
Chemical Abstracts Nomenclature (Collective Index Number);
(Registry Number)
Dimethyl carbonate:
Carbonic acid, dimethyl ester; (616-38-6)
Methyl 2-oxo-1,2,3,4-tetrahydronaphthalene-1-carboxylate:
1-Naphthalenecarboxylic acid, 1,2,3,4-tetrahydro-2-oxo-, methyl ester; (31202-23-0)
Sodium hydride; (7646-69-7)
β-Tetralone:
2(1H)-Naphthalenone, 3,4-dihydro-; (530-93-8)
Diisopropylamine:
2-Propanamine, N-(1-methylethyl)-; (108-18-9)
Butyllithium; (109-72-8)
Sodium iodide; (7681-82-5)
Sodium azide; (26628-22-8)
3-(3'-Azidopropyl)-3,4-dihydronaphthalen-2(1H)-one:
2(1H)-Naphthalenone, 3-(3-azidopropyl)-3,4-dihydro-; (137125-35-0)
Trifluoroacetic acid; (76-05-1)
2,3,11,11a-Tetrahydro-1H-benzo[d]pyrrolo[1,2-a]azepin-5(6H)-one:
5H-Pyrrolo[2,1-b][3]benzazepin-5-one, 1,2,3,6,11,11a-hexahydro-; (137125-3
7-2)
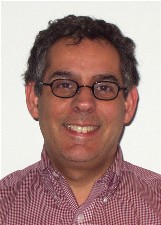 |
Born in the late 50s, Jeff Aubé attended the University of Miami, where he did undergraduate research with Professor Robert Gawley. He received his Ph.D. in chemistry in 1984 from Duke University, working with Professor Steven Baldwin, and was subsequently an NIH postdoctoral fellow at Yale University with Professor Samuel Danishefsky. In 1986, he moved to the University of Kansas, where he is now Professor of Medicinal Chemistry. Aubé's work involves the development of new synthetic reactions and their use in the synthesis of interesting natural products or for library generation.
|
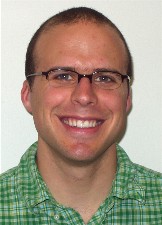 |
Scott Grecian was born in 1979, in Jamestown, ND. He attended Walla Walla University and received his B.S. in 2002. In the fall of 2002 he enrolled in the Department of Medicinal Chemistry at The University of Kansas and worked injoined the research group of Jeff Aubé, receiving his Ph.D. in 2007. His graduate research involveds the investigation of double conjugate addition reactionssequences and their application of such reactions in toward the formaltotal synthesis of the alkaloid natural product gelesemine.
|
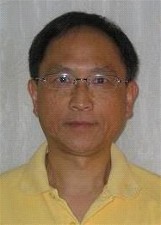 |
Dalian Zhao was born in Shanghai, China and obtained his B.S. degree in Chemical Engineering from East China Institute of Chemical Engineering in 1982. He came to the United States in 1987 and earned a M.S. degree in Organic Chemistry in 1989 from the University of North Texas. He joined Merck & Co., Inc. Process Research, (Rahway, New Jersey) in 1989. He received his Ph.D. in Organic Chemistry from Rutgers University (New Brunswick) in 2000 under Professor Spenser Knapp. He is currently a Senior Research Chemist, responsible for the discovery and development of efficient and environmentally safe multistep processes, providing active pharmaceutical ingredients from gram to multi kilo scale.
|
Copyright © 1921-, Organic Syntheses, Inc. All Rights Reserved