Org. Synth. 2008, 85, 27
DOI: 10.15227/orgsyn.085.0027
FACILE SYNTHESIS OF 2-ETHYL-3-QUINOLINECARBOXYLIC ACID HYDROCHLORIDE
Submitted by Brian R. McNaughton and Benjamin L. Miller
1.
Checked by Daniel P. Parker and John A. Ragan.
1. Procedure
A. 2-Ethyl-3-Quinolinecarboxylic Acid Methyl Ester. A flame-dried, 1000-mL, three-necked, round-bottomed flask is equipped with a Teflon-coated magnetic stirbar, internal thermometer, glass stopper, and a reflux condenser fitted with a nitrogen inlet. The flask is charged with 10 grams of 3Å molecular sieves (Note 1), 2-nitrobenzaldehyde (10.0 g, 66.2 mmol), methyl propionylacetate (8.30 mL, 66.2 mmol) (Note 2), and zinc (II) chloride (18.0 g, 132 mmol) (Note 3). To the flask is added 175 mL of anhydrous methanol (Note 4). The flask is flushed with a stream of nitrogen, and then, with stirring, heated to an internal temperature of 67 °C for 1 h with a heating mantle. Tin(II) chloride (62.7 g, 331 mmol) is then slowly added to the flask over five min in equal portions (Note 5). The flask is again flushed with a stream of nitrogen and the reaction mixture is stirred at 67 °C for 12 h. The solution is then allowed to cool to room temperature. To a 500-mL beaker is added potassium carbonate (45.7 g, 331 mmol), which is dissolved completely in 300 mL water. The reaction solution is made alkaline (pH approximately 8, as determined by pH paper) through the slow addition of the potassium carbonate solution (ca. 225 mL) while stirring (Note 6), resulting in a light orange slurry. To this slurry is added 200 mL of diethyl ether, and the complete mixture is then filtered through a Büchner funnel (Whatman filter paper, 125 mm diameter) (Note 7). An additional 75 mL of ether is added to rinse the reaction vessel, and subsequently passed through the funnel. After all the aqueous / ethereal solution has passed through the funnel, it is washed with ethyl ether (2 × 100 mL) (Note 8). The filter cake is then transferred to a 500-mL Erlenmeyer flask and slurried in 300 mL of ethyl acetate for 30 min before being filtered through a Büchner funnel (Whatman filter paper, 125 mm diameter). The filtrates are combined, transferred to a 2000-mL separatory funnel, where the aqueous layer is removed and the organic layer is washed with brine (100 mL). The organic phase is dried by the addition of magnesium sulfate (15 g), then is filtered and and the filter cake is rinsed with ethyl acetate (100 mL). The filtrate is concentrated by rotary evaporation (40 °C, 80 mmHg) in a 1000-mL round bottom flask to provide the methyl ester 1 as a yellow oil.
B. 2-Ethyl-3-Quinolinecarboxylic Acid. To the 1000-mL round bottom flask containing 1 is added a mixture of 100 mL of THF and 75 mL of 2 M LiOH (aq) and a football-shaped magnetic stir bar. The flask is capped with a rubber septum, vented with a needle, and stirred at room temperature for 12 h. The THF is then removed by rotary evaporation (Note 9) to provide an aqueous solution of the lithium carboxylate.
C. 2-Ethyl-3-Quinolinecarboxylic Acid Hydrochloride. The above solution is made acidic, (pH of 1 as determined by pH paper) through the addition of 50 mL of 12 N hydrochloric acid which results in a dense, yellow slurry which is gently stirred for 5 min. The susupension is chilled to 0 °C in an ice bath (internal temperature). A fritted glass funnel (60 mm) is fitted with filter paper, and the slurry is filtered with suction. The filter cake is washed with chilled (0 °C) HPLC grade ether (2 × 20 mL) (Note 10). The solids are transferred to a 100-mL, round-bottomed flask and placed on a vacuum line (22 °C, 0.2 mmHg) for 24 h to remove traces of water. The title compound is isolated as a light yellow solid 11.2-11.4 g (71-72%) (Notes 11, 12).
2. Notes
1.
The molecular sieves were washed thoroughly with methylene chloride to remove particulates and were then dried in a 200 °C oven for at least 24 h.
2.
2-Nitrobenzaldehyde (98%) and methyl propionylacetate (98%) were purchased from Aldrich Chemical Company, Inc. and were used as received.
3.
Zinc(II) chloride (97%) was purchased from Strem chemicals and was used as received. Zinc(II) chloride is hygroscopic; best results are observed when using fresh material, or material stored under desiccation. The use of stoichiometric or sub-stoichiometric reagent resulted in a notable decrease in desired product formation.
4.
Methanol (anhydrous, Sure-Seal) was obtained from Sigma-Aldrich.
5.
Tin (II) chloride (98%) was purchased from Strem chemicals and was used as received. Complete addition of tin(II) chloride in a single step results in an exotherm of approximately 7 °C, mandating the described slow addition. It was found that 5 roughly equivalent portions (ca. 12.5 g each) of tin(II) chloride were needed to obtain high yields of the desired product.
6.
Gas is evolved during this addition; care should be taken.
7.
The large amounts of inorganic salts and residue from sieves produced a solid layer, thereby slowing the rate of filtration. It was found that gentle agitation of this layer by means of a spatula provided a quicker filtration.
8.
Similar to Note 6, it was found that gentle agitation of the inorganic salt layer during the passing of diethyl ether provided the best extraction.
9.
Rotary evaporation was conducted under a reduced pressure of 20 mmHg and heated in a 40 °C water bath.
10.
HPLC grade diethyl ether (anhydrous) was obtained from J. T. Baker.
11.
The product exhibits the following physicochemical properties: mp 190-192 °C; IR (thin film) 3375, 2676, 2362, 1643, 1459, 1378, 1319, 1287, 1265, 1230 cm
−1;
1H NMR
pdf (400 MHz, D
2O) δ: 1.35 (t,
J = 7.7 Hz, 3 H), 3.41 (q,
J = 7.6 Hz, 2 H), 7.83 (t,
J = 7.5 Hz, 1 H), 8.02-8.10 (m, 2 H), 8.16 (d,
J = 8.3 Hz, 1 H), 9.33 (s, 1 H);
13C NMR
pdf (100 MHz, D
2O) δ: 13.6, 27.0, 119.5, 125.7, 126.6, 130.0, 130.2, 136.9, 138.3, 149.0, 162.5, 166.9; MS (Pos. ES): 202 (M+H, 100). Product purity was established by analytical HPLC: t
R, 2.98 min (70% (0.1% TFA in water) / 30% (0.1% TFA in acetonitrile) for 8 min followed by a gradient to 100% (0.1% TFA in acetonitrile) at 11.0 min, (Waters Symmetry C18 column (150 mm × 4.6 mm) 220 and 254 nm). At both wavelengths, only a single peak was observed, suggesting >99% product purity.
12.
Elemental analysis was problematic for establishing purity. The anhydrous formula (C
12H
12ClNO
2) requires: C, 60.64; H, 5.09; N, 5.89. The checkers found: C, 52.84; H, 5.52; N, 5.14. The bis-hydrate (C
12H
16ClNO
4) requires: C, 52.66; H, 5.89; N, 5.12. Thus, the analysis is consistent with the bis-hydrate. However, Karl-Fischer analysis indicated 10.8% water by weight, whereas the bis-hydrate requires 13.2% water. Based on this data the product is likely between a sesqui- (1.5) and bis- (2.0) hydrate.
Handling and Disposal of Hazardous Chemicals
The procedures in this article are intended for use only by persons with prior training in experimental organic chemistry. All hazardous materials should be handled using the standard procedures for work with chemicals described in references such as "Prudent Practices in the Laboratory" (The National Academies Press, Washington, D.C., 2011 www.nap.edu). All chemical waste should be disposed of in accordance with local regulations. For general guidelines for the management of chemical waste, see Chapter 8 of Prudent Practices.
These procedures must be conducted at one's own risk. Organic Syntheses, Inc., its Editors, and its Board of Directors do not warrant or guarantee the safety of individuals using these procedures and hereby disclaim any liability for any injuries or damages claimed to have resulted from or related in any way to the procedures herein.
3. Discussion
The quinoline skeleton is an important motif, and has been thought of as a “privileged” structure due to its presence in a broad array of natural products and pharmaceuticals.
1 Historically, quinoline-containing small molecules such as quinine and chloroquine have most notably been recognized as anti-malarial drugs.
2 However, more recently a variety of small molecules containing the quinoline substructure have been identified targeting a wide range of conditions.
3 This variant of the Friedländer synthesis
4 described above may be used for the generation of 2- and 3-substituted quinolines, from readily available starting materials. Unlike a variety of quinoline syntheses that call for the use of harsh acidic
5 or basic
6 reactants, this procedure provides the desired quinoline skeleton through the use of mild Lewis acidic and reducing conditions, and has been shown to proceed in the presence of a variety of functional groups.
7 In addition, the carboxylic acid moiety of the title compound allows for easy coupling through solid phase synthesis,
8 as well as further synthetic elaboration. The utility of this reaction is demonstrated in the table below.
Table 1. One-pot quinoline synthesis using a variety of substituted aryl aldehydes and symmetric ketones.
Appendix
Chemical Abstracts Nomenclature (Collective Index Number);
(Registry Number)
2-Nitrobenzaldehyde; (552-89-6)
Methyl propionylacetate: Pentanoic acid, 3-oxo-, methyl ester; (30414-53-0)
Zinc (II) chloride; (7646-85-7)
Tin (II) chloride; (7772-99-8)
2-Ethyl-3-Quinolinecarboxylic acid, methyl ester (119449-61-5)
2-Ethyl-3-Quinolinecarboxylic acid (888069-31-6)
2-Ethyl-3-Quinolinecarboxylic acid hydrochloride (888014-11-7)
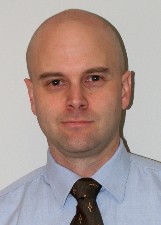 |
Benjamin L. Miller was born in 1967 in Dayton, Ohio. He carried out his undergraduate studies at Miami University (Ohio), receiving degrees in Chemistry, Mathematics, and German in 1988. From there Ben moved to Stanford University, earning a Ph. D. in Chemistry under the direction of Paul Wender, and then to Harvard University, as an NIH postdoctoral fellow in the laboratory of Stuart Schreiber. Ben joined the faculty of the University of Rochester in 1996, where he is currently Associate Professor of Dermatology, Biochemistry and Biophysics, and Biomedical Engineering. Research in his group includes dynamic combinatorial chemistry, molecular recognition, and biomedical nanotechnology, with a particular focus on biosensing. |
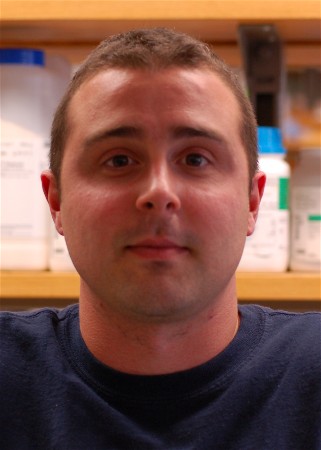 |
Brian R. McNaughton was born in Alexandria, Virginia. He attended Indiana University of Pennsylvania, graduating with a Bachelors of Science degree in 2001. He came to the University of Rochester in the Fall of 2002 and began graduate studies in Chemistry. His thesis research under the direction of Professor Benjamin L. Miller focused on the synthesis and evaluation of static and dynamic combinatorial libraries, as well as the development of novel methods in dynamic combinatorial chemistry. In 2004, he received a Master of Science degree from the University of Rochester. Following completion of his Ph.D. in 2007, he began postdoctoral research under the direction of Professor David R. Liu at Harvard University. |
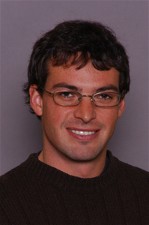 |
Daniel Parker was born in 1976 in St. Paul, Minnesota. He earned a B.S. degree in chemistry at the State University of West Georgia. He then joined Prof. Tarek Sammakia's lab at the University of Colorado, receiving his M.S. degree in 2004. He currently works as a process chemist in the Chemical Research and Development group at Pfizer in Groton, Connecticut. |
Copyright © 1921-, Organic Syntheses, Inc. All Rights Reserved