Org. Synth. 2008, 85, 34
DOI: 10.15227/orgsyn.085.0034
PREPARATION OF A NON-SYMMETRICAL IMIDAZOLIUM SALT: 1-ADAMANTYL-3-MESITYL-4,5-DIMETHYLIMIDAZOLIUM TETRAFLUOROBORATE
Submitted by Alois Fürstner, Manuel Alcarazo, Vincent César, and Helga Krause
1.
Checked by Chad Hopkins and Peter Wipf
2.
1. Procedure
A. 3-(Mesitylamino)butan-2-one. A 250-mL, single-necked round-bottomed flask equipped with a Teflon-coated magnetic stir bar is charged with 3-hydroxybutan-2-one (13.2 g, 150 mmol, 1.5 equiv), mesitylamine (13.52 g, 100 mmol, 1.0 equiv) (Note 1), toluene (150 mL) (Notes 2 and 3) and a catalytic amount of concentrated HCl (approximately 0.05 mL). The flask is then fitted with a Dean-Stark trap topped with a reflux condenser fitted with a nitrogen inlet. The solution is heated at reflux in a silicon oil bath for 3 h and the water generated is collected in the Dean-Stark trap (ca. 1.5 mL). The resulting yellow solution is allowed to cool to ambient temperature and the solvent is removed by rotary evaporation (25 °C, 8 mmHg). The oily residue is transferred to a 50-mL, one-necked, round bottomed flask and is purified by short path distillation (Note 4) under high vacuum (3 × 10−2 mmHg) to give 16.72 g (81%) of 3-(mesitylamino)butan-2-one as a pale yellow oil, bp 94-98 °C (Notes 5, 6, and 7).
B. N-Mesityl-N-(3-oxobutan-2-yl)formamide. An oven-dried, 100-mL, three-necked, round-bottomed flask equipped with two rubber septa, a nitrogen inlet and a Teflon-coated stir bar is charged with 3-(mesitylamino)butan-2-one (16.4 g, 80 mmol, 1.0 equiv) and anhydrous THF (25 mL) (Notes 3 and 8). Acetic formic anhydride (10.6 g, 120 mmol, 1.5 equiv) (Note 9) is added in one portion to this solution, and the resulting mixture is stirred at ambient temperature for 22.5 h (Note 10). The resulting brown mixture is transferred to a 100-mL, one-necked flask and is concentrated by rotary evaporation (25 °C, 8 mmHg) to a volume of approximately 20 mL before it is added on top of a wet-packed silica gel column (diameter: 7 cm, height: 22 cm, pre-treated with hexanes/EtOAc, 4:1) (Note 11). The product is eluted with hexanes/EtOAc, 3:1) to give 14.34 g (77%) of N-mesityl-N-(3-oxobutan-2-yl)formamide as a white, crystalline solid (Notes 12 and 13).
C. 1-Adamantyl-3-mesityl-4,5-dimethylimidazolium tetrafluoroborate. A 500-mL, three-necked, round-bottomed flask equipped with a pressure-equalizing dropping funnel, a nitrogen inlet, an internal thermometer, and a Teflon-coated stir bar (length: 4 cm) is charged with N-mesityl-N-(3-oxobutan-2-yl)formamide (13.7 g, 59 mmol, 1.0 equiv) and acetic anhydride (59 mL, 622 mmol, 10.6 equiv) (Note 1). The flask is immersed in an ice/water bath before aqueous HBF4 (48% w/w, 7.53 mL, 59.3 mmol, 1.01 equiv) (Note 1) is added dropwise through the addition funnel over a period of approximately 5 min to the stirred reaction mixture (Note 14). Once the addition is complete, the resulting brown solution is stirred at ambient temperature for 3 h. Anhydrous Et2O (400 mL) (Notes 3 and 8) is then added, leading to the separation of 5-acetoxy-3-mesityl-4,5-dimethyl-oxazolinium tetrafluoroborate as a pale brown oil. The supernatant organic layer is carefully decanted and the residual oil is rinsed twice with Et2O (2 × 200 mL). The remaining viscous oil is then suspended in toluene (100 mL) (Note 2). 1-Adamantylamine (11.54 g, 76.3 mmol, 1.3 equiv) (Note 1) is added and the resulting mixture is vigorously stirred at ambient temperature for 3 h. Addition of anhydrous Et2O (200 mL) (Notes 3 and 8) again causes the formation of two phases. The top Et2O layer is discarded and the viscous bottom phase is carefully rinsed with anhydrous Et2O (2 × 200 mL) to remove excess adamantylamine. The resulting pale yellow semi-solid is suspended in toluene (150 mL) (Note 2) and then acetic anhydride (16.6 mL, 176.1 mmol, 3.0 equiv) (Note 1) and aq. HBF4 (48% w/w, 2.24 mL, 17.6 mmol, 0.3 equiv) (Note 1) are introduced. The dropping funnel and internal thermometer are then replaced by a reflux condenser and a glass stopper and the mixture is stirred and heated to reflux in a silicon oil bath for 22 h (Note 15). After reaching ambient temperature, the mixture is transferred to a one-necked flask, the solvent is removed by rotary evaporation (25 °C, 8 mmHg), the resulting brown syrup is triturated with anhydrous Et2O (3 × 75 mL or until the ether layer is colorless) (Notes 3 and 8), and the ether layers are discarded. The resulting white solid product is collected and dried under vacuum (0.2 mmHg) to give 14.87 g (58%) of pure 1-adamantyl-3-mesityl-4,5-dimethylimidazolium tetrafluoroborate (Notes 16, 17, and 18). Crystals for elemental analysis are obtained by dissolving a sample of the product in a minimum amount of CH2Cl2 (Note 2) and careful layering of the resulting solution with anhydrous Et2O (Et2O/CH2Cl2, 7:1) (Notes 3 and 8). The resulting solution is kept at ambient temperature for 3 d, resulting in the precipitation of 1-adamantyl-3-mesityl-4,5-dimethyl-imidazolium tetrafluoroborate as colorless prisms which are collected and dried under vacuum (0.2 mmHg).
2. Notes
1.
3-Hydroxybutan-2-one (97%),
mesitylamine (98%),
aq. HBF4 (Alfa Aesar),
1-adamantylamine (97%, Aldrich Chemical Company, Inc.), and
acetic anhydride (ACS certified, Fisher) were used as received.
2.
Although the submitters took great care to ensure anhydrous conditions
(Note 3), the checkers found this precaution unnecessary.
3.
The submitters utilized purified solvents by distillation over the indicated drying agents prior to use and transferred them under Ar: THF, Et
2O (Mg-anthracene), hexanes, toluene (Na/K), CH
2Cl
2 (CaH
2). Reagent grade EtOAc (Fluka) was used as received. All reaction flasks were oven dried.
4.
The dimensions of the short path still are as follows: Jacketed, single piece construction with inlet for vacuum/inert gas, 10/18 thermometer joint at the top of the head, 14/20 joints for distillation and collection flasks, approx. 105 mm width (length of condenser) × 105 mm height (head).
5.
Working at 50% scale, the checkers obtained 8.93 g (87%).
6.
This product slowly decomposes on standing at ambient temperature and should be used in the next step without undue delay. It can be stored at -20 °C under Ar for several days.
7.
The product has the following physicochemical properties:
1H NMR
pdf (600 MHz, CDCl
3) δ: 1.23 (d,
J = 7.2 Hz, 3 H), 2.20 (s, 3 H), 2.22 (s, 3 H), 2.26 (s, 6 H), 3.96 (br s, 1 H), 4.04 (q,
J = 7.2 Hz, 1 H), 6.80 (s, 2 H);
13C NMR
pdf (150 MHz, CDCl
3) δ: 18.2, 18.8, 20.4, 27.4, 61.2, 129.0, 129.5, 130.8, 141.5, 209.9; IR (film) 3373, 2971, 2919, 1717, 1594, 1485, 1445, 1356, 1235, 1158, 1127, 1012, 855 cm
−1; HRMS (EI)
m/z calcd for C
13H
19NO 205.1467, found 205.1469. Anal. Calcd for C
13H
19NO: C, 76.06; H, 9.33; N, 6.82. Found: C, 76.26; H, 9.51; N, 6.78.
8.
Anhydrous THF (99.9%, Acros),
anhydrous Et2O (ACS certified, Fisher) toluene (ACS certified, Fisher),
hexanes (ACS certified, Fisher),
ethyl acetate (ACS certified, Fisher),
dichloromethane (ACS certified, Fisher),
chloroform (stabilized with ca. 1% ethanol, Acros) were used as received.
9.
Acetic formic anhydride is conveniently prepared according to: Krimen, L. I.
Org. Synth.
1970,
50, 1-3.
10.
Although TLC monitoring occasionally shows small amounts of unreacted starting material after 24 h reaction time, prolonged stirring does not improve the yields. TLC monitoring is performed with precoated plates with silica gel 60 F
254 purchased from E. Merck, Darmstadt using hexanes/EtOAc, 3:1 as the eluent; R
f (substrate) = 0.73; R
f (product) = 0.36.
11.
Silica gel 32-63 D (60 Å) (MP Biomedicals, Germany, purchased through Bodman Industries, Aston, PA) was used.
12.
Working at 50% scale, the checkers obtained 8.06 g (80%).
13.
The product has the following physicochemical properties: mp 100-101 °C;
1H NMR
pdf (600 MHz, CDCl
3) δ: 0.98 (d,
J = 7.2 Hz, 3 H), 2.14 (s, 3 H), 2.30 (s, 3 H), 2.38 (s, 3 H), 2.40 (s, 3 H), 4.52 (q,
J = 7.2 Hz, 1 H), 6.91 (s, 1 H), 6.96 (s, 1 H), 7.99 (s, 1 H);
13C NMR
pdf (150 MHz, CDCl
3) δ: 13.5, 18.4, 18.7, 20.9, 27.7, 59.4, 59.4, 129.0, 129.7, 133.2, 137.8, 138.7, 138.8, 163.6, 205.3; IR (KBr) 2987, 2916, 1720, 1653, 1484, 1452, 1318, 1296, 1252, 1166, 1029 cm
−1; HRMS (ESI+)
m/z calcd for C
14H
19NO
2Na 256.1313, found 256.1317; Anal. Calcd for C
14H
19NO
2: C 72.07, H 8.21, N 6.00, found C 72.04, H 8.26, N 5.98.
14.
The addition was carried out at a rate such that an internal temperature of 27 °C was not exceeded.
15.
The submitters stated that the reaction requires 40-60 h for completion; however, the checkers observed completion of the reaction after 22 h based on TLC analysis. The progress of the reaction can be followed by TLC (precoated plates with silica gel 60 F
254 purchased from E. Merck, Darmstadt) using CHCl
3/MeOH, 20:1 as the eluent: R
f (product) = 0.58.
16.
The submitters obtained a pale grey solid (15.2-17.0 g, 60-67%) by suspending the crude product in anhydrous Et
2O
(Note 3) and immersing the suspension in an ultrasound cleaning bath (Sonorex RK SIOH) for 20 min.
17.
Working at 50% scale, the checkers obtained 7.99 g (62%).
18.
The product has the following physicochemical properties: mp 238-241 °C (dec.);
1H NMR
pdf (600 MHz, CDCl
3) δ: 1.79 (q,
J = 12.6 Hz, 6 H), 1.94 (s, 3 H), 1.96 (s, 6 H), 2.33 (s, 6 H), 2.34 (s, 6 H), 2.61 (s, 3 H), 7.00 (s, 2 H), 8.46 (s, 1 H);
13C NMR
pdf (150 MHz, CDCl
3) δ: 8.1, 12.8, 17.3, 21.1, 29.6, 35.2, 41.2, 63.4, 63.4, 127.2, 129.0, 129.5, 129.8, 132.9, 134.9, 141.1; IR (KBr) 3183, 2916, 2856, 1609, 1543, 1456, 1308, 1240, 1208, 1050, 903, 856, 806, 737 cm
−1; HRMS (ESI+)
m/z calcd for C
24H
33N
2 349.2644, found 349.2610; Anal. Calcd for C
24H
33BF
4N
2: C, 66.06; H, 7.62; N, 6.42. Found: C, 66.29; H, 7.69; N, 6.43.
Handling and Disposal of Hazardous Chemicals
The procedures in this article are intended for use only by persons with prior training in experimental organic chemistry. All hazardous materials should be handled using the standard procedures for work with chemicals described in references such as "Prudent Practices in the Laboratory" (The National Academies Press, Washington, D.C., 2011 www.nap.edu). All chemical waste should be disposed of in accordance with local regulations. For general guidelines for the management of chemical waste, see Chapter 8 of Prudent Practices.
These procedures must be conducted at one's own risk. Organic Syntheses, Inc., its Editors, and its Board of Directors do not warrant or guarantee the safety of individuals using these procedures and hereby disclaim any liability for any injuries or damages claimed to have resulted from or related in any way to the procedures herein.
3. Discussion
N-Heterocyclic carbenes of type
A have gained a prominent role as ancillary ligands for a host of transition metal templates in various oxidation states
3 and as organocatalysts in their own right.
4 They are usually prepared by deprotonation of the corresponding imidazolium salts
B with a non-nucleophilic base (Scheme 1).
Although this tremendous success story has led to a huge number of structural variants, it is surprising that several obvious and seemingly trivial substitution patterns have not been investigated in any great detail. For example, unsymmetrical imidazolium salts bearing two different aryl groups on their N-atoms are largely unknown. Likewise, non-symmetrical imidazolium salts bearing one
N-aryl and one
N-alkyl group are rare and essentially limited to those having primary
N-alkyl substituents.
5 These gaps in the structural landscape reflect the inherent limitations of the established syntheses routes which largely rely on
N-substitution reactions of appropriate imidazole precursors. While such
N-substitutions work well with reactive halides R
2−X,
6 they are difficult to accomplish or even impossible with aryl halides,
tert-alkyl halides and many
sec-alkyl halides, in particular if they are chiral and non-racemic.
Scheme 1. Formation of imidazolium salts B and N-heterocyclic carbenes A: established N-substitution methodology (left) versus the heterocycle interconversion strategy (right).
Outlined in the procedure described above is an alternative approach based on a heterocycle interconversion strategy which provides ready access to substitution patterns beyond the reach of customary methodology.
7 Specifically, readily available
N-formamido carbonyl derivatives
F are cyclized to the corresponding oxazolinium salts
E (Z = O, Y = OAc), which react with an amine of choice R
2NH
2 to give hydroxylated imidazolinium salts
D (Z = NR
2, Y = OH) as the primary products. This condensation can be achieved with variously substituted anilines as well as secondary and tertiary amines, even if they are poorly nucleophilic and/or sterically hindered. Compounds
D are then dehydrated to the desired imidazolium salts
B on exposure to Ac
2O and catalytic amounts of a suitable mineral acid.
This novel methodology is distinguished by the following characteristics:
7
- As mentioned above, a host of different amines R2NH2 is accommodated, thus giving access to non-symmetrical imidazolium salts B that are inaccessible or, at least, very difficult to make otherwise. The examples outlined in the text and in Figure 1 fall into this category.
Figure 1. Selected non-symmetrical imidazolium salts prepared by the heterocycle interconversion strategy.
- The intermediates D and E need not be rigorously purified; the conversion of the N-formamido carbonyl derivatives F into the desired imidazolium salts B is therefore straightforward and operationally highly convenient.
- This largely streamlined protocol, the good to excellent overall yields, and a practical workup procedure greatly facilitate the scale-up of the method. In many cases, the final products can be readily isolated by simple precipitation with Et2O, ultrasonication of the crude product, and filtration of the resulting salts.
- Because the imidazolium salts derive from three simple building blocks, the method is inherently flexible and allows for structural variations of every substituent on the backbone of the final products. Moreover, proper choice of the mineral acid in the cyclization step F → E allows different escorting counterions to be chosen.
- Enantiopure amines are not racemized and various chiral products, including bicyclic constructs, are also within reach (Figure 1).
This user-friendly methodology therefore nicely complements the existing routes to imidazolium salts and the
N-heterocyclic carbenes derived thereof.
7 Because of its practicality and inherent flexibility, it is expected to foster further applications of such ligands and catalysts in advanced organic synthesis.
Appendix
Chemical Abstracts Nomenclature (Collective Index Number);
(Registry Number)
3-Hydroxybutan-2-one; (513-86-0)
Mesitylamine: Benzenamine, 2,4,6-trimethyl-; (88-05-1)
3-(Mesitylamino)butan-2-one: 2-Butanone, 3-[(2,4,6-trimethylphenyl)-amino]-; (898552-96-0)
Acetic formic anhydride; (2258-42-6)
N-Mesityl-N-(3-oxobutan-2-yl)formamide: Formamide, N-(1-methyl-2-oxopropyl)-N-(2,4,6-trimethylphenyl)-; (898553-01-0)
Acetic anhydride; (108-24-7)
Aqueous HBF4, Borate(1-), tetrafluoro-, hydrogen (1:1): (16872-11-0)
1-Adamantylamine: Tricyclo[3.3.1.13,7]decan-1-amine; (768-94-5)
1-Adamantyl-3-mesityl-4,5-dimethylimidazolium tetrafluor
oborate
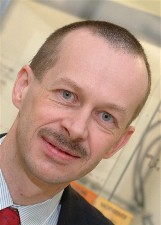 |
Alois Fürstner was born in Bruck/Mur, Austria, in 1962. He obtained his training and PhD degree from the Technical University of Graz, Austria, working on carbohydrates under the supervision of Hans Weidmann. After postdoctoral studies with the late Prof. W. Oppolzer in Geneva, he finished his Habilitation in Graz before joining the Max-Planck-Institut für Kohlenforschung, Mülheim/Ruhr, Germany, in 1993, where he is presently one of the Directors. His research interests focus on homogeneous catalysis, metathesis, and organometallic chemistry as applied to natural product synthesis. |
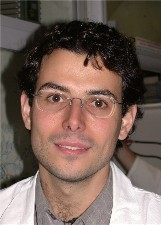 |
Manuel Alcarazo was born in 1978 in Alcalá de Guadaira, Spain. After graduating from the he University of Sevilla in 2000, he obtained his PhD in 2005 at the Instituto de Investigaciones Químicas (CSIC-USe) under the supervision of Dr. José M. Lassaletta. There he worked on the synthesis and applications of N-heterocyclic carbenes derived from hydrazines. He is currently a postdoctoral associate at Prof. A. Fürstner's research group at the Max-Plank-Institut für Kohlenforschung involved in various projects ranging from ligand design to natural product total synthesis. |
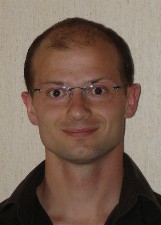 |
Vincent César was born in 1977 in Nancy, France. He was educated at the Ecole Normale Supérieure in Lyon. He joined the group of Prof. Lutz H. Gade at Université Louis Pasteur to obtain his doctorate in 2004 studying a new class of oxazolinyl-imidazolylidene ligands for catalysis. In 2004 he joined the group of Prof Dr. Alois Fürstner (Max Planck Institute für Kohlenforschung, Mülheim/Ruhr) as a postdoctoral fellow. He is currently a Chargé de Recherche at the Laboratoire de Chimie de Coordination of CNRS in Toulouse focusing on the catalytic activation and functionalization of unreactive C-H bonds. |
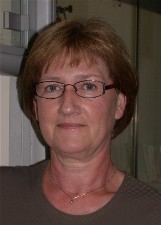 |
Helga Krause was born in 1952 in Oberhausen, Germany. She started her education in 1969 as a laboratory assistant at the Max-Planck-Institute for Coal Research. After completing these studies in 1972 she worked in the group of Professor Roland Köster in boron-organic chemistry. In 1994 she joined the group of Professor Alois Fürstner working on various projects in the field of organic and organometallic chemistry. |
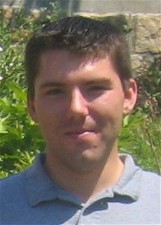 |
Chad Hopkins obtained his B.Sc. (2001) from Mississippi State University. He then moved to the University of Kansas to pursue his Ph.D. with Professor Helena Malinakova, focusing on the development of novel palladium-catalyzed multi-component coupling and cascade processes for the synthesis of highly substituted homoallylic alcohols, amines, d-lactones, and unnatural amino acids. After completion of his Ph.D. in 2007, he joined the group of Professor Peter Wipf at the University of Pittsburgh as a postdoctoral research associate and is currently working on the synthesis of new PLK1 inhibitors as well as the natural product disorazole C1. |
Copyright © 1921-, Organic Syntheses, Inc. All Rights Reserved