Org. Synth. 2009, 86, 1
DOI: 10.15227/orgsyn.086.0001
PREPARATION OF (3,5-DIMETHOXY-1-PHENYL-CYCLOHEXA-2,5-DIENYL)-ACETONITRILE THROUGH BIRCH REDUCTIVE ALKYLATION (BRA)
Submitted by Raphaël Lebeuf, Muriel Berlande, Frédéric Robert, and Yannick Landais
1.
Checked by Marcus G. Schrems and Andreas Pfaltz.
1. Procedure
Caution! The Birch reductive alkylation reaction (part B) should be carried out in a well-ventilated hood to avoid exposure to ammonia.
A. 5-Phenyl-1,3-dimethoxybenzene. To a 250-mL, three-necked, round-bottomed flask, equipped with a magnetic stirring bar, a reflux condenser (central neck), an internal thermometer and a ground-glass stopper, 5-phenylcyclohexane-1,3-dione (7.98 g, 42.4 mmol) (Note 1) and methanol (80 mL) are added (Note 2). Concentrated sulfuric acid (1 mL, 95%) is added dropwise over 1 min and the suspension turns into a homogeneous solution. After 10 min iodine (23.7 g, 93.4 mmol, 2.20 equiv) is added as a solid in one portion. The mixture is refluxed for 45 min (oil bath; T = 85 °C) and then cooled down to room temperature. The solution is added to an aqueous sodium thiosulfate solution (0.5 m, 350 mL) and 150 mL of ether. Using a 1-L separatory funnel the aqueous phase is separated and the organic phase is washed with 2 × 100 mL of 0.5 m sodium thiosulfate solution, giving a yellowish organic phase. The aqueous phases are combined and extracted with 2 × 150 mL of ether. The combined organic phase was washed with brine (50 mL), dried over sodium sulfate (1.80 g) and concentrated on a rotary evaporator (600 mmHg, 40 °C, then 15 mmHg), (8.87 g, light brown oil). Silica gel (10 g) and ethyl acetate (50 mL) are added and agitated. The solvent is removed on a rotary evaporator (125 mmHg, 40 °C, then 15 mmHg). A sintered glass funnel (h × d: 9 cm × 5 cm) is charged with 20 g of silica gel (ca. 2.5 cm layer). A layer of sand (0.5 cm) is put on top followed by the crude material adsorbed on silica gel and another layer of sand (0.5 cm). A mixture of hexanes and ethyl acetate (50:1; 750 mL) is passed through the silica gel plug by applying vacuum from below and collected (Note 3). After evaporation of the solvent on a rotary evaporator and drying under vacuum (0.1 mmHg) biaryl 1 is obtained as a white solid (6.04-6.42 g, 66-71%, mp 61.0-61.9 °C) (Note 4).
B. (3,5-Dimethoxy-1-phenyl-cyclohexa-2,5-dienyl)-acetonitrile. A 500-mL, three-necked, round-bottomed flask is equipped with a magnetic stirring bar. The central neck of the reaction flask is fitted with a large cold-finger condenser. The flask with the condenser is heated in an oven overnight (120 °C). The two remaining necks are closed with ground-glass stoppers and the hot glassware is connected via the cold-finger condenser to a vacuum-nitrogen line. Vacuum is applied until the glassware is cooled down to room temperature and the glassware is flushed with nitrogen (Note 5). Biaryl 1 (6.08 g, 28.4 mmol) is introduced under nitrogen, and dissolved in anhydrous THF (100 mL) (Note 6). A T-joint with a bubbler for exhaust is introduced between the vacuum-nitrogen line and the cold-finger condenser. One ground-glass stopper is exchanged with a gas inlet valve connected to an ammonia tank (Note 7, 8) the other stopper with an internal thermometer. The flask and the cold-finger condenser are cooled to -78 °C (bath temperature) using acetone and dry-ice, then ammonia (200 mL approximately) is condensed (Note 9). The gas inlet is replaced with a stopper and lithium (435 mg, 62.7 mmol, 2.21 equiv) (Note 10) is added portion wise at -78 °C under a nitrogen flow during 10 min. During lithium addition the solution turns rapidly brown and finally brick red. After 45 minutes of stirring at this temperature, one of the glass stoppers is replaced by a septum and a cold solution of chloroacetonitrile (5.40 mL, 85.3 mmol, 3.00 equiv) in anhydrous THF (20 mL) is added dropwise over 10 min, keeping the temperature below -70 °C (Note 11). The mixture turns immediately brown. After 20 min of stirring, the reaction is quenched by addition of solid ammonium chloride (3.50 g). The cooling bath and condenser are removed and ammonia is allowed to evaporate under air (Note 12). When the reaction mixture has reached room temperature, a saturated ammonium chloride solution (150 mL) is added, followed by water (50 mL). The aqueous phase is extracted with ether (3 × 100 mL) in a 1-L separating funnel. The combined organic phases are washed with brine (150 mL), dried over sodium sulfate (2 g) and concentrated on a rotary evaporator (125 mmHg, 40 °C, then 15 mmHg). The resulting brown paste (8.20 g) is purified by flash chromatography (Note 13). After concentration of the product-containing fractions on a rotary evaporator and drying under vacuum (0.1 mmHg), the bis-enol ether is obtained in 2 fractions: white solid (5.11 g) with mp = 101.0-102.0 °C (Note 14), off-white fraction (1.17 g) with mp = 98.0-99.0 °C (Note 15) (total yield: 6.28-6.65 g, 87-88%).
2. Notes
1.
5-Phenylcyclohexa-1,3-dione (97%) was purchased from Alfa-Aesar and was used as received.
2.
Distillation over magnesium prior to use did not improve the yield.
Sulfuric acid (ACS grade 95-98%) was purchased from Alfa-Aesar,
sodium thiosulfate anhydrous was purchased from SDS,
ether (99%) was purchased from SDS,
ammonium chloride (99%) was purchased from Alfa-Aesar. The Checkers used methanol from J. T. BAKER (Baker analyzed), ammonium chloride from Fluka (≥99%), iodine from Riedel-de-Haën (purris. p.a.), sulfuric acid from VWR (AnalR NORMAPUR, 95%), sodium thiosulfate pentahydrate from Sigma-Aldrich (≥99.5%), ether form J. T. BAKER (Baker analyzed, distilled prior to use).
3.
The Submitters used column chromatography for purification of the product: silica gel column (25 × 4.5 cm charged with 100 g of silica gel Si60 43-60 μm, purchased from Merck), eluted with 1 L of a 95/5 petroleum/EtOAc mixture. The product has an R
f = 0.82 when eluting with a 90/10 petroleum ether/EtOAc mixture. Checkers: The product has a R
f = 0.42 when eluting with a 90/10 hexanes/EtOAc mixture. The purity (elemental analysis,
1H NMR) of the material purified by column chromatography and by filtration over silica gel (Procedure A) was identical.
4.
Analytical data closely matched published data
2:
1H NMR
pdf (400.1 MHz, CDCl
3) δ: 3.86 ppm (s, 6 H), 6.49 (t, 1 H,
J = 2.2 Hz), 6.75 (d, 2 H,
J = 2.3 Hz), 7.34-7.38 (m, 1 H), 7.42-7.46 (m, 2 H), 7.58-7.60 (m, 2 H).
13C NMR
pdf (100.6 MHz, CDCl
3) δ: 55.4, 99.2, 105.4, 127.2, 127.5, 128.7, 141.2, 143.5, 161.0. IR (ATR) 3085, 3009, 2970, 2939, 2839, 1589, 1450, 1411, 1350, 1311, 1204, 1150, 1064, 1026, 926, 833, 764, 694, 656 cm
-1. MS (EI, 70 eV)
m/
z (%): 214 (M
+, 100), 185 (20), 128 (13). Anal Calcd for C
14H
14O
2: C, 78.48; H, 6.59. Found: C, 78.34; H, 6.66.
5.
Submitters: All connections and septa were secured with Parafilm-M
®. The Checkers used GLINDEMANN
®-sealing rings (PTFE) purchased from AMSI-Glas AG.
6.
The Checkers used THF (VWR, HPLC-grade) dried using a Pure-Solv
TM system.
11
7.
Anhydrous ammonia (N36) was purchased from Air Liquide in a steel cylinder (6 bar, 9.6 m
3). The Checkers used anhydrous ammonia form PanGas in a steel cylinder (10 L, 5.3 kg).
8.
A drying tower filled with potassium hydroxide pellets is placed between the cylinder and the flask in order to dry the ammonia.
9.
30-45 minutes are usually required to condense ammonia. An estimation of the volume of condensed ammonia was carried out by graduating the reaction flask with 300 mL of a solvent, prior to the experiment.
10.
Lithium wire, purchased from Aldrich (99.9%, 0.01% Na) is cut and washed in a beaker filled with dry pentane or cyclohexane. After weighing out the required amount, the wire is cut into 8-10 pieces in the beaker. Each piece is then hammered in an aluminum foil covered with mineral oil in order to protect the lithium surface from air and moisture. The pieces have to be flattened thoroughly to ensure rapid reaction. Each shiny flattened piece is finally washed quickly with dry pentane and introduced immediately into the reaction mixture under nitrogen atmosphere.
11.
Three equivalents of electrophile are required as lithium amide (LiNH
2) is formed during the reaction that can consume part of the electrophile. Chloroacetonitrile (>99% GC) was purchased from Fluka and used as received. The chloroacetonitrile solution is prepared in a dry 50-mL Schlenk tube (sealed with a septum) under nitrogen and is cooled down to -78 °C before addition via cannula. A nitrogen filled balloon is used for pressure regulation.
12.
The fume hood should be pulled down during evaporation of ammonia. A water bath may be used to accelerate the evaporation.
13.
The crude mixture was adsorbed on silica gel (10 g) before being loaded at the top of a silica gel column (h × d: 19 cm × 4 cm, 120 g silica gel 43-60 μm, purchased from Merck). The fractions were collected in 50 mL tubes. The column is eluted with 800 mL of a 90/10 hexanes/EtOAc mixture, then 1 L of a 50/10 hexanes/EtOAc mixture and 1.2 L of a 30/10 hexanes/EtOAc mixture. A first fraction (tubes 32-43) contained 5.11 g of a colorless solid, mp 101-102 °C, a second fraction (tubes 44-60) contained 1.17 g of an off-white solid mp 98-99 °C
(Note 15), the product has a R
f = 0.13 when eluting with a 90/10 hexanes/EtOAc mixture.
14.
Analytical data of
2:
1H NMR
pdf (400.1 MHz, CDCl
3)δ: 2.87 (dt, 1 H,
J = 20.7, 1.2 Hz), 2.93 (s, 2 H), 2.97 (dt, 1 H,
J = 20.7, 1.2 Hz), 3.60 (s, 6 H), 4.70 (t, 2 H,
J = 1.2 Hz), 7.22-7.27 (m, 1 H), 7.34-7.35 (m, 4 H).
13C NMR
pdf (100.6 MHz, CDCl
3) δ: 31.1, 32.2, 44.9, 54.5, 54.5, 98.4, 118.0, 125.7, 126.8, 128.6, 146.3, 153.3. IR (ATR) 3057, 2996, 2960, 2929, 2899, 2828, 2252, 1694, 1406, 1209, 1196, 1164, 1146, 1022, 870, 809, 766, 703 cm
-1. MS (FAB)
m/
z (%) 256 ([M+H]
+, 26), 215 (100). HRMS [M+Na]
+ C
16H
17NNaO
2: Calcd 278.1157; found: 278.1160. Anal Calcd for C
16H
17NO
2: C, 75.27; H, 6.71; N, 5.49; found: C, 75.50; H, 6.82; N, 5.32.
15.
The compound collected as a second fraction showed no impurities in the
1H NMR spectrum. However, elemental analysis was outside the accepted range. Anal Calcd for C
16H
17NO
2: C, 75.27; H, 6.71; N, 5.49; found: C, 74.86; H, 6.74; N, 5.74.
Handling and Disposal of Hazardous Chemicals
The procedures in this article are intended for use only by persons with prior training in experimental organic chemistry. All hazardous materials should be handled using the standard procedures for work with chemicals described in references such as "Prudent Practices in the Laboratory" (The National Academies Press, Washington, D.C., 2011 www.nap.edu). All chemical waste should be disposed of in accordance with local regulations. For general guidelines for the management of chemical waste, see Chapter 8 of Prudent Practices.
These procedures must be conducted at one's own risk. Organic Syntheses, Inc., its Editors, and its Board of Directors do not warrant or guarantee the safety of individuals using these procedures and hereby disclaim any liability for any injuries or damages claimed to have resulted from or related in any way to the procedures herein.
3. Discussion
Birch reductive alkylation (BRA)
3 has proven to be a valuable process for the synthesis of highly functionalized building blocks, including cyclohexadienes bearing a quaternary center. The strategy is well documented on aryls containing electron-withdrawing groups and this strategy was elegantly extended by Schultz
4 to diastereoselective reductive alkylation of chiral amides. More recently, Donohoe devised a Birch reductive alkylation of heteroarenes (furan, pyrrole, and pyridine) bearing electron-withdrawing groups using an ammonia-free protocol.
5 Pioneering studies
6 on Birch reductive alkylation of biaryls showed that mixture of compounds with modest level of regioselectivities and yields were obtained depending on the nature of the substituents on both aromatic rings. Recent investigations in our laboratory, however, demonstrated that BRA on biaryls bearing electron-donating groups (OMe) could be a very efficient process, affording good to excellent yields of alkylated products.
7 Minor variations on the nature and the number of substituents on the aromatic rings were effectively shown to strongly affect both the regiocontrol and the competition between protonation and alkylation. It was, however, possible to control the regioselectivity of the reduction with a careful choice of the nature of the substituents on the biaryls. Two methoxy groups on the first aromatic ring,
meta to the biaryl linkage, were thus shown to activate the reduction of this ring. Substituents such as OH or NHR on the second ring, in any positions relative to the biaryl bond, were found to be compatible with this reduction, providing their acidic proton was removed with
n-BuLi, prior to addition of Li and ammonia. In contrast, a methoxy group placed in
ortho or
para position was cleaved under these conditions. The resulting substituted cyclohexa-1,4-dienes are valuable intermediates for the synthesis of more elaborated targets and were used as starting materials for the synthesis of various alkaloids using desymmetrization processes.
8
The above procedure is representative of the synthesis of diversely substituted arylcyclohexa-1,4-dienes. The strategy also features a convenient preparation of biaryls having a 3,5-dimethoxyphenyl moiety starting from a cyclohexa-1,3-dione
9, adapted from published protocols,
10 which has been found, on large scale, more straightforward and practical than palladium couplings. The Birch reductive alkylation follows a standard protocol and can be run on large scale without erosion of the yield, in general. This methodology has been successfully extended to others biaryls with minor modifications, as shown in Table 1.
Table 1
Appendix
Chemical Abstracts Nomenclature (Collective Index Number);
(Registry Number)
5-Phenylcyclohexane-1,3-dione (493-72-1)
Iodine (7553-56-2)
Sodium thiosulfate (7772-98-7)
Lithium (7439-93-2)
Chloroacetonitrile (107-14-2)
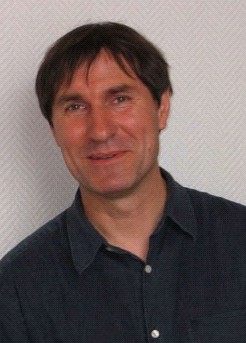 |
Yannick Landais was born in Angers (France) in 1962. He received his Ph.D. in chemistry from the University of Orsay (Paris XI) under the supervision of Dr. J.-P. Robin. After carrying out postdoctoral work with Prof. Ian Fleming at Cambridge University (1988-1990), he took a position of Assistant Professor at the University of Lausanne (1990-1997). He was then appointed at the University Bordeaux 1 where he is currently Professor of Organic Chemistry. His research interests are in asymmetric synthesis, radical chemistry, with a special emphasis on organosilicon chemistry and its applications in total synthesis of natural products. In 1997, he was awarded the Werner prize by the New Swiss Chemical Society.
|
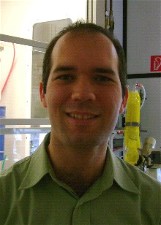 |
Raphaël Lebeuf was born in 1980 in Carcassonne. He did all his undergraduate studies at the University Bordeaux 1 and started research in 2002 under the supervision of Prof. Y. Landais and Dr. F. Robert. During his Ph.D. studies, he worked on Birch reductive alkylations of biaryls and desymmetrization including hydroamination processes. After graduating from the University Bordeaux 1 in 2006, he accepted a postdoctoral position in Prof. Glorius group at Münster University (Germany).
|
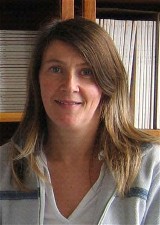 |
Muriel Berlande was born in Saint-Etienne (France) in 1969. She studied chemistry at the University of Clermont-Ferrand where she did her Master of chemistry in 1994 under the supervision of Prof J. C. Gramain. She was then appointed as a laboratory assistant at the University Bordeaux 1 in 1997 and finally laboratory technician at the CNRS in 2000. She is currently involved in different multi-step organic syntheses and specialized in HPLC analysis.
|
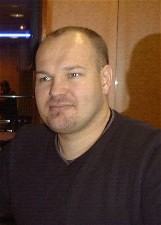 |
Frédéric Robert studied in Grenoble where he received his Ph.D. in 1999 under the supervision of Dr. Andrew E. Greene and Dr. Yves Gimbert. He moved to Dartmouth College (New Hampshire, USA) for a postdoctoral study with Prof. Peter A. Jacobi (2000-2001), followed by a second postdoctorate at the University of Geneva with Prof. Peter Kündig (2001-2002). He was appointed Chargé de Recherches CNRS at the University Bordeaux 1 (2002). His current research focuses on the development of new desymmetrization processes for alkaloid synthesis.
|
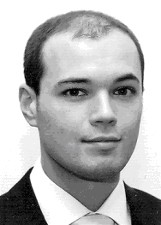 |
Marcus G. Schrems was born in Groß-Umstadt (Germany) in 1979. He studied chemistry at the Technische Universität München (TUM), National University of Singapore (NUS) and Universtiy of Bergen (Norway) and graduated from TUM in 2005 after completing his Diplom-Thesis under the direction of R. Anwander and W. A. Herrmann. In 2006 he joined the lab of Andreas Pfaltz at University of Basel (Switzerland). He is currently working on Ir-catalyzed enantioselective hydrogenation of unfunctionalized olefins, focusing on tetrasubstituted olefins.
|
Copyright © 1921-, Organic Syntheses, Inc. All Rights Reserved