Org. Synth. 2010, 87, 184
DOI: 10.15227/orgsyn.087.0184
A GENERAL METHOD FOR COPPER-CATALYZED ARYLATION OF ACIDIC ARENE C-H BONDS. PREPARATION OF 2-CHLORO-5-(3-METHYLPHENYL)-THIOPHENE
Submitted by Joseph Alvarado, Hien-Quang Do, and Olafs Daugulis
1.
Checked by Peter C. Marsden and Jonathan A. Ellman.
1. Procedure
A 50-mL oven dried Schlenk flask with a 14/20 joint equipped with a magnetic stir bar is charged with 2-chlorothiophene (7.11 g, 60.0 mmol, 2.0 equiv) and 1-iodo-3-methylbenzene (6.54 g, 30.0 mmol, 1.0 equiv). The Schlenk flask is equipped with a 14/20 thermometer adaptor, a thermometer is inserted through the adaptor into the flask so that the bulb is completely submerged in the solution, the adaptor is then tightened so that the o-ring holds the thermometer in place, and finally the joint is wrapped tightly with Teflon tape. The flask is evacuated and back filled with dry nitrogen gas three times through the side arm. After placing it inside the glove box (Note 1), the thermometer adaptor is removed from the joint, and the flask is charged with lithium t-butoxide (4.80 g, 60.0 mmol, 2.0 equiv), 1,10-phenanthroline (1.08 g, 6.00 mmol, 0.20 equiv), CuI (1.14 g, 6.00 mmol, 0.20 equiv), and DMPU (18 mL; tetrahydro-1,3-dimethyl-2(1H)-pyrimidinone) (Note 2). The flask is re-equipped with the thermometer and adapter, which is again wrapped tightly with Teflon tape. The Schlenk flask is taken out of the glove box. A hose attached to a Schlenk line is purged with dry nitrogen gas and connected to the side arm of the Schlenk flask. The stopcock valve is opened and the Schlenk flask is evacuated and back filled with dry nitrogen gas three times through the side arm stopcock. The flask is placed in a preheated oil bath at 125 °C and magnetically stirred for 12 h (Note 3). The brown color of the reaction mixture changes to deep red after 5-10 min and then to dark brown 30 min later.
After the reaction is complete as judged by GC analysis (Note 4), the reaction mixture is allowed to cool to room temperature. At that point, nitrogen atmosphere is not required any further. After dilution with EtOAc (30
mL) and stirring for 1 min, the reaction mixture is transferred to a 500 mL Erlenmeyer flask. The Schlenk flask is washed with EtOAc (3 × 50 mL), the EtOAc solutions are combined, and brine (100
mL) is added. The resulting mixture contains a light brown precipitate that is removed via vacuum filtration by using a 7 cm diameter filter funnel with a medium porosity fritted disk. The brown precipitate is washed with EtOAc (3 × 10 mL), and the resulting filtrate is transferred to a 500 mL separatory funnel. The top organic layer is separated from the bottom aqueous layer. The aqueous layer is extracted with additional ethyl acetate (3 × 100 mL) (Note 5). The combined organic layers are dried over MgSO4 (Note 6; about 9 g) followed by vacuum filtration into a 1000 mL round bottom flask by using a 7 cm diameter filter funnel with a medium porosity fritted disk. The filtrate is concentrated by rotary evaporation (50 °C, 30 mm Hg) to give 8-10 mL of crude product. The crude product is purified by flash chromatography on SiO2 (Note 7), using hexanes as the eluent (4.8 L) and collecting 100 mL fractions. The first 12 fractions are blank (1.2 L), followed by 6 fractions containing starting material and minor amounts of impurities (0.6 L), and then pure product for 30 fractions (3 L collected). After concentration of the fractions containing the pure product by rotary evaporation (30 °C, 30 mmHg), 5.73 g (92%) of 2-chloro-5-(3-methylphenyl)-thiophene is obtained as an air stable, light yellow oil that crystallizes when cooled to -20 °C (Notes 8 and 9; mp 38-39 °C).
2. Notes
1.
In addition to the procedure reported above, the checkers also performed a half-scale reaction outside of the glovebox where all of the starting materials were quickly measured out in a fumehood, and then transferred to the reaction flask. The Schlenk flask was equipped with a 14/20 thermometer adaptor, a thermometer was inserted through the adaptor into the flask so that the bulb was submerged in the solution, the adaptor was then tightened so that the o-ring held the thermometer in place, and finally the joint was wrapped tightly with Teflon tape. The flask was then evacuated and backfilled with nitrogen via the sidearm stopcock. Heating, work-up and purification remained unchanged from above and 2.70 g (86%) of product was obtained. LiO
tBu should not be stored outside of an inert atmosphere because it is very hygroscopic.
2.
All reagents were used as received. The submitters obtained 2-chlorothiophene (98%) from Matrix Scientific, 1-iodo-3-methylbenzene (99%) from Oakwood Products, Inc., lithium
t-butoxide (98+%) from Strem Chemicals, 1,10-phenanthroline (99+%) and CuI (98+%) from Acros Organics, and DMPU (tetrahydro-1,3-dimethyl-2(1H)-pyrimidinone, 98+%) from Alfa Aesar. Lithium
t-butoxide and CuI were stored in an argon-filled glove box. The checkers obtained 2-chlorothiophene (98%) from TCI America, 1-iodo-3-methylbenzene (99%) from Aldrich, lithium
t-butoxide (98+%) from Strem Chemicals, 1,10-phenanthroline (99+%) and CuI (98+%) from Acros Organics, and DMPU (tetrahydro-1,3-dimethyl-2(1H)-pyrimidinone, (98+%) from Alfa Aesar.
3.
Stir bar size: 2.5 cm length and 1 cm diameter. Stirring rate 1000-1100 rpm.
4.
GC analyses (by the submitters) were performed on a Shimadzu CG-2010 chromatograph equipped with a Restek column (Rtx®-5, 15 m, 0.25 mm ID). The following program parameters were used: initial temperature: 80 °C (2 min), ramp at 35 °C/min to 250 °C, hold at 250 °C for 3 min. Retention times: 2-chlorothiophene 0.77 min, 1-iodo-3-methylbenzene 2.92 min, and 2-chloro-5-
m-tolylthiophene 5.27 min. GC analyses (by the checkers) were performed on an Agilent 6890N chromatograph equipped with an Agilent column (Ultra 2, crosslinked 5% Ph Me Silicone, 25 m × 0.2 mm × 0.33 μm film thickness). The program parameters specified by the submitters gave the following retention times: 2-chlorothiophene 2.49 min, 1-iodo-3-methylbenzene 4.87 min, and 2-chloro-5-
m-tolylthiophene 7.50 min. Aliquots from the reaction mixture were diluted with 1 mL of EtOAc and filtered for GC analysis. After 12 h, >98% of 1-iodo-3-methylbenzene was consumed.
5.
Organic and inorganic layers must separate completely. There must be a sharp border between the two. Otherwise, the yield will be lower.
6.
The submitters obtained magnesium sulfate (anhydrous powder) from Mallinckrodt Baker, Inc. The checkers obtained magnesium sulfate (anhydrous powder) from Fisher Chemical.
7.
The submitters performed flash chromatography on 60Å silica gel (Sorbent Technologies) with 460 g of SiO
2 in a 38 cm × 8 cm column equipped with a 500 mL reservoir. The checkers performed flash chromatography on 60 Å silica gel (MP Silitech 32-63D). All solvents were HPLC grade purchased from Fisher Chemical. The product has an R
f = 0.59 (hexanes; visualization by UV).
8.
The characterization of the product is as follows: FT-IR (neat,) 1601, 1488, 1445, 1214, 1022, 796, 779, 687 cm
-1;
1H NMR
pdf (500 MHz, CDCl
3) δ: 2.37 (s, 3 H), 6.86 (d,
J = 3.9 Hz, 1 H), 7.04 (d,
J = 3.9 Hz, 1 H), 7.10 (d,
J = 7.3 Hz, 1 H), 7.23-7.27 (m, 1 H), 7.29-7.31 (m, 2 H);
13C NMR
pdf (125 MHz, CDCl
3) δ: 21.5, 122.1, 122.7, 126.3, 127.1, 128.7, 128.92, 128.93, 133.6, 138.7, 143.1; Anal. calcd. for C
11H
9ClS: C, 63.30 H, 4.35; found: C, 62.96 H, 4.28.
9.
On half-scale, the checkers also obtained an 88% yield. On full scale, the submitters reported product yields of 5.68-5.81 g (90-93%).
Safety and Waste Disposal Information
All hazardous materials should be handled and disposed of in accordance with "Prudent Practices in the Laboratory"; National Academy Press; Washington, DC, 1995.
3. Discussion
The combination of copper iodide with phenanthroline ligand is a general catalyst for the arylation of acidic sp
2 carbon-hydrogen bonds.
2 It has been shown that electron-rich and electron-poor heterocycles as well as arenes possessing at least two electron-withdrawing groups can be arylated. The prerequisite for a successful arylation is an sp
2 C-H bond possessing a p
Ka of 35 or less (in DMSO).
3 The regioselectivity of arylation is very high, with the most acidic carbon-hydrogen bond being functionalized. A comparison between copper and palladium catalysis in C-H bond arylation is given below. The advantages of copper relative to palladium catalysis are as follows: (1) cheaper catalyst; (2) very predictable arylation regioselectivity - the most acidic C-H bond is always arylated; (3) nearly uniform reaction conditions due to simple mechanistic picture; (4) unusual regioselectivity - the most hindered C-H bond is arylated. The advantages of palladium relative to copper catalysis are: (1) possibility to use aryl chlorides as coupling partners,
4 while only ArBr, ArI, and most reactive heteroaryl chlorides can be used for copper catalysis; (2) ability to effect the arylation of less acidic C-H bonds such as nitrobenzene or indole;
5 (3) C-H bonds can be arylated in the presence of acidic N-H groups; in the case of copper catalysis, NH substituents will be arylated preferentially.
6 A mechanistically distinct procedure allows indole arylation by iodonium salts under copper catalysis.
7
An overview of substrates that can be arylated by employing copper catalysis is given in Table 1
2 Typically, 10 mol % of catalyst loading can be employed, although the above procedure was checked with a 20 mol % catalyst loading.
2 Electron-rich heterocycles such as thiazole, caffeine, and triazole can be arylated by aryl iodides and aryl bromides (entries 1-3). Electron-poor heterocycles such as 2-phenylpyridine oxide and pyridazine are also reactive (entries 4-5). Polyfluorobenzenes can be arylated in good yields (entries 6-7). Alkenylation is possible by employing alkenyl bromides (entry 8). Other electron-poor arenes such as polychloro-, nitro-, and cyanoarenes can be arylated at the most acidic position, which is also the most hindered one. For relatively non-acidic 1,3-dichlorobenzene, use of a hindered, strong Et
3COLi base is required to achieve reasonable product yield. For the most acidic compounds possessing p
Ka values of 27 or less (in DMSO), potassium phosphate base can be used. Other substrates require the use of
t-BuOLi base. Arylations by aryl bromides are successful only if K
3PO
4 is employed and low conversions are observed with
t-BuOLi base.
The reaction mechanism most likely involves an acid-base reaction followed by a copper-catalyzed carbon-carbon bond formation (Scheme 1). Both
t-BuOCu and
t-BuOLi were shown to be competent bases for the deprotonation step.
2 The deprotonation step determines overall arylation regioselectivity.
Scheme 1. Mechanistic considerations
Table 1. Arylation of Acidic sp2 C-H Bondsa
Appendix
Chemical Abstracts Nomenclature (Collective Index Number);
(Registry Number)
1-Iodo-3-methylbenzene; (625-95-6)
2-Chlorothiophene; (96-43-5)
Lithium t-butoxide; (1907-33-1)
1,10-Phenanthroline; (66-71-7)
Copper(I) iodide; (7681-65-4)
Tetrahydro-1,3-dimethyl-2(1H)-pyrimidinone; (7226-23-5)
2-Chloro-5-(3-methylphenyl)-thiophene; (1078 144-58-7)
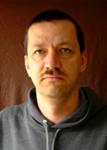 |
Olafs Daugulis was born in Riga, Latvia in 1968. He obtained his degree in chemical engineering from Riga Technical University in 1991. His PhD research was performed at the University of Wisconsin-Madison in the group of Prof. E. Vedejs. After obtaining the PhD in 1999 he joined the group of Prof. M. Brookhart at UNC-Chapel Hill as a postdoctoral associate. He is currently an Associate Professor of Chemistry at the University of Houston. |
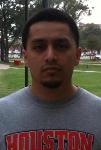 |
Joseph Alvarado was born in Houston, Texas in 1985. He entered University of Houston in 2004 where he is currently an undergraduate chemistry major. He plans to attend graduate school in chemistry. |
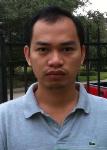 |
Hien-Quang Do was born in 1978 in Trà Vinh, Vietnam. He received his B.S. in chemistry from the University of Natural Sciences at Ho Chi Minh City in Vietnam. A few years after obtaining a Masters degree from the same University in 2003, he came to the University of Houston where he currently is a fourth-year graduate student in Prof. Daugulis' group. |
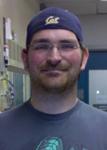 |
Peter Marsden was born in 1984 in Pasadena, CA. He obtained his B.A. in Chemistry from Pomona College in 2006. He is currently a 4th year graduate student working with Profs. Jonathan Ellman and Robert Bergman on creating commodity chemicals from biomass. |
Copyright © 1921-, Organic Syntheses, Inc. All Rights Reserved