Org. Synth. 2010, 87, 192
DOI: 10.15227/orgsyn.087.0192
1,3,5-TRIACETYLBENZENE
Submitted by Peter J. Alaimo
1, Amanda-Lynn Marshall, David M. Andrews, and Joseph M. Langenhan
1.
Checked by Liang Huang, Eric A. Bercot, and Margaret Faul.
1. Procedure
A single-necked, 250-mL round-bottomed flask equipped with a Teflon-coated magnetic stir bar (33 × 15 mm, egg-shaped) is charged with trans-4-methoxy-3-buten-2-one (15.3 mL, 15.0 g, 0.135 mol, 1.0 equiv), ethanol (120
mL) (Note 1), and deionized water (30
mL) (Note 1) under an atmosphere of air. Glacial acetic acid (17.5 M, 0.9 mL, 0.944 g, 0.015 mol, 0.11 equiv) (Note 1) is added via syringe to the reaction mixture. A reflux condenser is fitted to the round-bottomed flask and the resulting homogeneous pale yellow reaction mixture is heated gently to reflux, with stirring, for 48 h ensuring that the temperature of the oil bath is kept at 77 °C. Over this time, the reaction remains homogeneous, and turns orange in color (Note 2). The oil bath is removed, and the reaction flask is allowed to cool to room temperature, and then placed in a -20 °C freezer for 18 h to crystallize the product. The resulting mixture is then vacuum filtered while cold through a sintered-glass funnel (Note 3) to collect the yellow needles (Note 4), which are rinsed with pre-cooled (0 °C) anhydrous diethyl ether (4 × 50 mL) (Notes 1, 5 and 6). The crude product (6.4-6.6 g; 70-72%; mp = 161-165 °C) is recrystallized by dissolving the yellow needles in hot ethanol (200 mL, 78 °C), allowing the solution to cool to room temperature, then storing it in a -20 °C freezer for 18 h. The resulting mixture is then vacuum filtered while cold, through a sintered-glass funnel (Note 3), and the pale yellow needles are rinsed with pre-cooled (0 °C) anhydrous ether (4 × 50 mL) (Note 7). The 1,3,5-triacetylbenzene obtained is air dried overnight to provide 5.6-5.9 g of product (61-64%) as pale yellow needles (mp 163-165 °C) (Notes 8 and 9).
2. Notes
1.
The submitters purchased
trans-4-methoxy-3-buten-2-one (tech. grade, 90%) and ethanol (200 proof; HPLC grade) from Aldrich and used as received. The submitters purchased glacial acetic acid (Baker; 99%, 17.5
M) and diethyl ether (Baker; 99%, anhydrous) from VWR and used as received. The checkers used deionized water from a house system (municipal water is purified by reverse osmosis using a commercial system manufactured by US Filters). The checkers analyzed
trans-4-methoxy-3-buten-2-one (tech. grade, 90%) from Aldrich by
1H NMR versus phenylmethyl sulfone (99 % purity, Acros cat # 353040050) and found the material to be 90 wt %. All product yields are adjusted based on the assay of starting
trans-4-methoxy-3-buten-2-one. The instrument details for
1H NMR analysis are as follows: 400 MHz magnet, Bruker Avance II console, 5 mm BBFO probe, and a standard Bruker parameter set (zg30) with d1 set to 30 s.
2.
Reaction progress is monitored by thin layer chromatography (TLC) analysis using Whatman silica gel 60 F
254 analytical plates and 25% ethyl acetate/hexanes. Visualization is accomplished using 254 nm UV light and
p-anisaldehyde stain [prepared by dissolving
p-anisaldehyde (15g) in
EtOH (250) and slowly adding concentrated
H2SO4 (2.5 mL)]. R
f(
trans-4-methoxy-3-buten-2-one; tech. grade) = 0.26 (pink) and 0.35 (purple). R
f (1,3,5-triacetylbenzene) = 0.20 (light orange).
3.
The funnel used is a 150 mL medium-porosity 6 cm sintered-glass funnel purchased from Chemglass.
4.
The crude yellow needles are 1,3,5-triacetylbenzene.
5.
The filtrate (the combined supernatant and ether wash) is concentrated by rotary evaporation (34 °C, 20 mmHg) and analyzed by
1H NMR spectroscopy. Any 1,3,5-triacetylbenzene present may be recovered according to the same crystallization procedure described above to give 90-120 mg of additional 1,3,5-triacetylbenzene in approximately 80% purity (
1H NMR; diagnostic resonances at 8.70 and 2.71 ppm).
6.
The checkers carried out an analysis of the crude isolated product by
1H NMR and found the material to be 99 wt % versus phenylmethyl sulfone (99 % purity, Acros cat # 353040050) as an internal standard.
7.
Alternatively, purification of the crude product can be accomplished by sublimation. The crude yellow needles are placed in a water-cooled vacuum sublimator, which is then evacuated (0.01 mmHg), and heated to 110 °C for 12 h to give 0.90-0.95 g of product (as pale yellow crystals) per gram of starting crude yellow needles. The material obtained is spectroscopically indistinguishable (
1H NMR,
13C NMR, IR, GC-MS) from the pale yellow needles obtained from recrystallization from ethanol. Anal. calcd. for C
12H
12O
3: C, 70.56; H 5.93; found C, 70.50; H, 5.93. An advantage of the sublimation method is that it reduces the solvent waste by half, relative to recrystallization.
8.
Analytical data for the product are as follows: pale yellow needles; mp 162-165 °C; IR (ATR): 3358 (m), 3086 (m), 3064 (m), 3003 (m), 2924 (m), 1845 (w), 1683 (s), 1586 (m), 1418 (s), 1359 (s), 1316 (m), 1221 (s), 1089 (w), 1020 (w), 976 (m), 957 (w), 941 (w), 905 (m) cm
-1;
1H NMR
pdf (400 MHz, CDCl
3) δ: 2.72 (s, 9 H), 8.71 (s, 3 H);
13C NMR
pdf (100 MHz, CDCl
3) δ: 26.9, 131.7, 137.9, 196.6; HRMS (ESI)
m/z calcd. for C
12H
13O
3 ([M+H]
+) 205.0859; found 205.0857; GCMS (EI): 204 (M
+), 189 ([M-CH
3]
+), 161 ([M-C(O)CH
3]
+), 118 ([M-(C(O)CH
3)
2]
+), 75 (M-(C(O)CH
3)
3]
+); Anal. calcd. for C
12H
12O
3: C, 70.56; H 5.93; found: C, 70.45; H, 5.84. GCMS analysis was performed using an Agilent 6890 GC with 5973 MS detector. Helium was used as carrier gas at 1 mL/min and a column from Restek (Rtx-5MS, 30 m × 0.25 mm, 0.5 μm film thickness). Splitless injection; Injector: 250 °C; transfer line 280 °C; source 150 °C. Initial T: 50 °C for 0.5 min then ramp 15 °C/min to 300 °C, isothermal for 4 min. The retention time for the product was 13.86 min, and no additional signals were present. The checkers did not analyze the isolated product by GC/MS.
9.
Performing this procedure on smaller scale using 0.5 g of
trans-4-methoxy-3-buten-2-one consistently gives a 70-89% yield of recrystallized 1,3,5-triacetylbenzene; however, on a larger scale than reported herein reproducibly leads only to a 61-64% yield of recrystallized 1,3,5-triacetylbenzene, despite extensive optimization. The product obtained in the small scale reaction is spectroscopically indistinguishable (
1H NMR,
13C NMR) from the pale yellow needles obtained in the preparation described.
Safety and Waste Disposal Information All hazardous materials should be handled and disposed of in accordance with "Prudent Practices in the Laboratory"; National Academy Press; Washington, DC, 1995.
3. Discussion
1,3,5-Triacetylbenzene is a valuable material that has been used in the synthesis of materials such as dendrimers,
2 phenolic molecular glasses,
3 and cyanine dyes.
4 It has found use as a starting material for the synthesis of cyclophanes
5 and several ligands for transition metals;
6 and it is a commonly used probe for investigations of reaction mechanism and scope.
7
Several syntheses of 1,3,5-triacetylbenzene have been documented, including one in
Organic Syntheses (
Coll. Vol. 3, p.829 (1955); Vol. 27, p.91 (1947)). The procedure previously published in
Organic Synthesis involves the synthesis of the sodium salt of acetoacetaldehyde and its subsequent condensation in the presence of acetic acid to yield 1,3,5-triacetylbenzene in 30-38% yield (Scheme 1).
8 The method presented herein is preferred because it is more efficient (54-60% yield of analytically pure material), it is more convenient (reflux commercially available materials for 2 d), and it generates a small volume of less hazardous waste (250 mL of reaction waste that is a mixture of 120 mL of ethanol, 30 mL of distilled water, 0.9 mL of acetic acid, and 200 mL of diethyl ether). The recrystallization generates 400 mL of additional solvent waste consisting of 200 mL of ether and 200 mL of ethanol, but sublimation can be performed instead
(Note 6). The approximate cost of running this reaction, excluding solvents (ethanol, water, acetic acid, diethyl ether), is $38.
Scheme 1
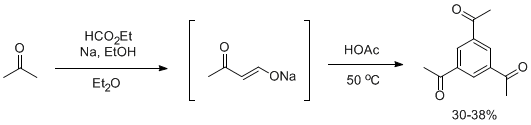
Seven methods have been reported previously for the synthesis of 1,3,5-triacetylbenzene. Three of these methods use transition metal catalysts. For example, the Eaton lab
9 employed cobalt(I) catalysts (Scheme 2A) in an overall [2+2+2] cyclotrimerization reaction of butyn-2-one to form 1,3,5-triacetylbenzene in 44% isolated yield. The Cadierno lab
10 used a ruthenium(IV) catalyst (Scheme 2B) in a similar cyclotrimerization reaction to generate a mixture (88:12) of 1,3,5-triacetylbenzene and 1,2,4- triacetylbenzene. Due to the expense of butyn-2-one (10 mL for $449.50; Aldrich), the method presented herein is preferable. Jiang and co-workers found that PdCl
2 catalyzes the cyclotrimerization of buten-2-one to give 1,3,5-triacetylbenzene in 58% yield (Scheme 2C).
11 Although this reaction yield is similar to the reaction reported herein, our procedure avoids the use of palladium, and is simpler to perform (the procedure reported by Jiang requires an autoclave for the reaction and chromatography for the subsequent separation).
Scheme 2
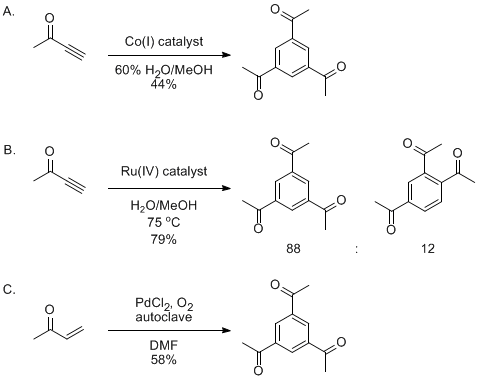
Four methods that do not employ transition metal catalysts have also been reported. For example, Shi and co-workers describe the reaction of a tribenzimidazolium salt with Grignard reagents to afford 1,3,5-triacetylbenzene (Scheme 3).
12 This multi-step procedure has the disadvantages of poor atom economy and yield (36%). Xue and co-workers reported a cyclotrimerization reaction in which 1,3,5-triacetylbenzene is formed by reacting butyn-2-one in the presence of 2,4-pentanedione and DMAP (Scheme 4A).
13 Although the reported yield is excellent (95%), the method requires chromatography and uses butyn-2-one, an expensive reagent (10 mL for $449.50; Aldrich), as the starting material. Lastly, the condensation of enaminones provides 1,3,5-triacetylbenzene, as described by Elnagdi and co-workers (Scheme 4B).
14 This procedure, which is very similar to the procedure presented herein, uses
trans-4-dimethylamino-3-buten-2-one (25 g for $185; Aldrich), which is roughly twice as expensive as
trans-4-methoxy-3-buten-2-one (50 g for $184; Aldrich).
Scheme 3
Scheme 4
In summary, the procedure presented herein is a simple process for the preparation and purification of 1,3,5-triacetylbenzene from trans-4-methoxy-3-buten-2-one. This method is advantageous relative to other synthetic strategies because the method is simple, requires no specialized equipment, is cost-effective, and generates a small quantity of waste that is relatively nontoxic.
Appendix
Chemical Abstracts Nomenclature (Collective Index Number);
(Registry Number)
trans-4-Methoxy-3-buten-2-one; (51731-17-0)
1,3,5-Triacetylbenzen e; (779-90-8)
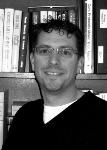 |
Peter J. Alaimo was born in 1968 in New York. He completed his undergraduate studies at the University of Michigan with Prof. Brian P. Coppola, and earned his Ph.D. at the University of California at Berkeley with Prof. Robert G. Bergman. He spent five years with Prof. Kevan M. Shokat (University of California at San Francisco) as a postdoctoral researcher. He began his independent career at Seattle University in 2004. His research interests include the development of new synthetic methods and asymmetric catalysts. |
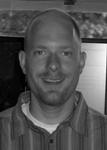 |
Joseph M. Langenhan completed his undergraduate degree at Allegheny College in 1998. He then pursued graduate studies with Professor Samuel H. Gellman at the University of Wisconsin-Madison's Department of Chemistry and earned a Ph.D. in Organic Chemistry in 2003. After two years of post- doctoral research with Professor Jon S. Thorson at the Laboratory for Biosynthetic Chemistry in the School of Pharmacy at the University of Wisconsin-Madison, he moved to Seattle University in 2005, where he is currently an Assistant Professor. He is currently developing chemoselective glycosylation methods for novel applications. |
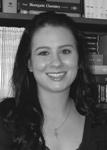 |
Amanda-Lynn Marshall was born in 1988 in Georgia. She completed her undergraduate studies at Seattle University where she earned her B.S. in General Science with concentrations in Chemistry and Biology. She was recently awarded a Rotary Ambassadorial Scholarship, which will allow her the opportunity to study abroad and obtain her M.Sc. in Clinical Microbiology/Immunology. After her studies abroad she plans to attend medical school. |
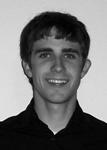 |
David M. Andrews was born in 1988 in Utah. He is currently enrolled in Seattle University's undergraduate program working towards a B.S. in General Science. He is researching the enantioselective synthesis of 2,3-dihydropyridin-4-ones under the direction of Professor Alaimo. After graduation he plans to attend graduate school. |
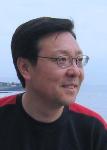 |
Liang Huang obtained his M.S. degree in Chemistry from Murray State University in 1997. He then began working at AlliedSignal as a synthetic organic chemist. In 1998 he moved to CB Research and Development, Inc. taking a position as Scientist. He has been working in Amgen's Chemical Process Research and Development group as a Process Chemist since 1999. |
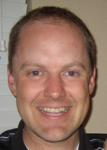 |
Eric A. Bercot completed his B.S. in Chemistry at the University of Oregon in 1999 while working in the laboratories of Michael M. Haley. He received his Ph.D. from Colorado State University in 2004 working with Professor Tomislav Rovis where he investigated the use of cyclic anhydrides as eletrophilic coupling partners in transition metal mediated cross-coupling reactions. In 2004, Eric joined the Chemical Process Research and Development group at Amgen where he is currently a Senior Scientist. |
Copyright © 1921-, Organic Syntheses, Inc. All Rights Reserved