Org. Synth. 2010, 87, 275
DOI: 10.15227/orgsyn.087.0275
SYNTHESIS OF ENAMIDES FROM KETONES: PREPARATION OF N-(3,4-DIHYDRONAPHTHALENE-1-YL)ACETAMIDE
Submitted by Hang Zhao
1, Charles P. Vandenbossche, Stefan G. Koenig, Surendra P. Singh
1, and Roger P. Bakale.
Checked by Gopal K. Datta and Jonathan A. Ellman.
1. Procedure
Caution! Triethylphosphine is extremely pyrophoric and has a strong odor. Care must be taken to avoid exposure to air at all times. The reagent, the reaction and its work-up should be handled in an adequately ventilated fume hood while wearing gloves, safety glasses and laboratory coat.
A. 3,4-Dihydronaphthalene-1(2H)-one oxime. An oven-dried 250-mL, three-necked, round-bottomed flask equipped with a reflux condenser, nitrogen inlet adapter, glass stopper, and magnetic stir bar (octagonal; 5/16 inch diameter; 1 inch length) is charged with α-tetralone (20.0 g, 137 mmol, 1.0 equiv) (Note 1), methanol (120 mL) (Note 2), hydroxylamine hydrochloride (10.5 g, 150 mmol, 1.1 equiv) (Note 3), and anhydrous sodium acetate (12.3 g, 150 mmol, 1.1 equiv) (Note 4) at room temperature. The flask is flushed with nitrogen, and the nitrogen inlet adapter is then moved to the top of the condenser. A temperature probe is placed into the third neck of the flask. The flask is heated at reflux in an oil bath. After one hour at reflux, the reaction is complete (Note 5). The mixture is cooled to room temperature, and methanol is removed on a rotary evaporator (2030 °C, 30 mmHg). The resulting slurry is re-dissolved in ethyl acetate (200 mL) (Note 6), and 2 N aqueous NaOH (60 mL) is added. The mixture is transferred to a separatory funnel (500 mL), and the organic phase is separated, washed with DI water (100 mL) and brine (100 mL, 15 wt%), and concentrated at reduced pressure on a rotary evaporator (3040 °C, ~30 mmHg) to give a brown crystalline solid (21.2 g, 96% yield) (Note 7) that was used in the next step without further purification.
B. N-(3,4-Dihydronaphthalene-1-yl)acetamide. An oven-dried 250-mL, three-necked round-bottomed flask equipped with a reflux condenser, thermometer, rubber septum, nitrogen inlet through rubber septum, and magnetic stir bar (octagonal; 5/16 inch diameter; 1 inch length) is charged with crude 3,4-dihydronaphthalen-1(2H)-one oxime (20.0 g, 124 mmol, 1.0 equiv) and toluene (freshly distilled and dry, 200 mL) (Note 8). The resulting solution is flushed with nitrogen for 50 min at room temperature. Triethylphosphine (Note 9) in a glass ampule is opened in a glove box, and the liquid triethylphosphine is securely transferred into a Schlenk flask (oven dried and cooled under nitrogen), sealed, and brought inside the fume hood. Triethylphosphine (17.6 g, 22.0 mL, 149.0 mmol, 1.2 equiv) is thereafter transferred into a properly marked volumetric container (oven dried and cooled under nitrogen) via cannula from the Schlenk flask under a nitrogen atmosphere (Figure 1). Triethylphosphine is then added directly to the reaction mixture from the volumetric container via cannula over 1 min (Figure 2). The reaction mixture is stirred at room temperature for 15 min. Acetic anhydride (15.2 g, 14.1 mL, 149.0 mmol, 1.2 equiv) is charged to the reaction using a syringe over 15 min (Note 10), which causes an exothermic reaction and the temperature rises to 39 °C (measured by an inserted thermometer immersed into the reaction mixture) (Figure 3). A yellow slurry solution (Note 11) is formed thereafter. The rubber septum and thermometer are quickly replaced with glass stoppers and the nitrogen inlet is moved to the top of the condenser. The resulting slurry is heated to reflux in an oil bath for 16 h, and reaction progress is monitored by HPLC or TLC (Notes 12 and 13). After complete conversion is achieved (Note 14), the reaction mixture is cooled to room temperature (~25 °C), 6 N aqueous NaOH (60 mL) and tetra-n-butylammonium hydroxide in MeOH (1 N, 2.0 mL) (Note 15) are added. The mixture is stirred at room temperature for 23 h until complete conversion of imide to enamide is achieved (Note 16). The reaction mixture is diluted with ethyl acetate (500 mL) and transferred to a 1-L separatory funnel, where the organic phase is separated, washed with 1 wt% acetic acid solution in water (100 mL), followed by brine (100 mL, 15 wt%) (Note 17).
Figure 1
Figure 2
Figure 3
The solution is then concentrated on a rotary evaporator (3040 °C, ~30 mmHg) to give a slurry (~80 g, ~85 mL) (Note 18). The slurry is then heated in an oil bath to 100 °C (oil bath temperature) to form a clear solution. The toluene solution is cooled to 90 °C and heptane (30 mL) (Note 19) is slowly charged to achieve a cloudy solution. The cloudy solution is stirred at 90 °C for 10 min, until a thin slurry is formed (Note 20). Additional heptane (70 mL) is charged over 5 min at 90 °C. The slurry is stirred at 90 °C for an additional 20 min and then cooled to room temperature over 2 h. After stirring at ambient temperature overnight (Note 21), the solid is collected by filtration on a Büchner funnel, washed with a mixture of 40% toluene and 60% heptanes (v/v, 2 x 40 mL), and dried under vacuum (45 °C at 15 mmHg for 5 h) to give an off-white crystalline solid (20.2 g, 87% yield) (Notes 22 and 23).
2. Notes
1.
α-Tetralone (97% purity) was purchased from Aldrich Chemical Company, Inc., and used as supplied.
2.
Methanol, HPLC grade, was purchased from EMD Chemicals, Inc., and used as supplied.
3.
Hydroxylamine hydrochloride was purchased from Aldrich Chemical Company, Inc., and used as supplied.
4.
Sodium acetate (99% purity) was purchased from Aldrich Chemical Company, Inc., and used as supplied.
5.
The progress of the reaction can be monitored by thin layer chromatography (TLC) on Multiformat (pre-scored to 5 x 20 cm) silica gel 60 F
254 plates from Merck KGaA, elution with 10:90 (
v/v) ethyl acetate/hexanes, and visualization with UV light. R
f values: tetralone, 0.40; oxime, 0.30. Alternatively, the reaction can also be monitored by HPLC. One drop of an aliquot was diluted with 1 mL of eluent and analyzed by: Conditions A (submitters), Waters 2695 HPLC system using a C18 SunFire column 3.5 µm, 4.6 x 150 mm; UV detection at 250 nm; eluent 40:60 of 0.1 vol% aqueous 85% phosphoric acid/acetonitrile, respectively at flow rate of 1 mL/min. Retention times for tetralone and oxime are 3.7 and 3.4 min, respectively: Conditions B (checkers), Hewlett Packard 1100 HPLC system using a Agilent narrow-bore ZORBAX SB-C18 column 5.0 µm, 2.1 x 150 mm; detection at 254 nm, 210 nm, 230 nm, and 280 nm ; eluent 95:5 of H
2O:acetonitrile (0.1 vol% TFA) at a flow rate of 0.4 mL/min. Retention time for tetralone and the oxime are 9.0 and 8.8 min, respectively. After 1 h at reflux, the sample showed >99.0 area% of oxime.
6.
Reagent grade ethyl acetate was purchased from EMD Chemicals, Inc., and used as supplied (submitters).
7.
The crude product displays the following physicochemical properties: Mp 100102 °C;
1H NMR
pdf (400 MHz, CDCl
3) δ: 1.84-1.91 (m, 2 H), 2.76 (t, 2 H,
J = 6.0 Hz), 2.84 (t, 2 H,
J = 6.7 Hz), 7.16 (d, 1 H,
J = 7.4 Hz), 7.187.28 (m, 2 H), 7.87 (d, 1 H,
J = 7.7 Hz);
13C NMR
pdf (100 MHz, CDCl
3) δ: 21.2, 23.9, 29.8, 124.0, 126.4, 128.6, 129.2, 130.4, 139.8, 155.3; HRMS (ESI)
m/z calcd. for C
10H
12NO ([M+H]
+) 162.0919; found 162.0912.
8.
Reagent grade
toluene was purchased from EMD Chemicals, Inc., and used as supplied (submitters).
9.
Triethylphosphine (97% purity) was purchased from Cytec Industries, Inc., and used as supplied (submitters), and in a glass ampule, purchased from Alfa Aesar, stock no: 30177, lot no: G25T018 (checkers). Triphenylphosphine is also effective in this transformation, but removal of the by-product, triphenylphosphine oxide, by flash chromatography, proved difficult at scale. For scales >1 g, we recommend using triethylphosphine which enables easy isolation of the enamide by crystallization from the reaction mixture after work up.
10.
Reagent grade
acetic anhydride was purchased from Aldrich Chemical Company, Inc., and used as supplied.
11.
A slight exotherm was observed with a temperature rise from 23 °C to 39 °C with formation of solid acylated oxime intermediate
(Note 12).
12.
The reaction can be monitored by HPLC analysis of a sample prepared by diluting one drop of the reaction mixture with 1 mL of eluent.. Retention times of the intermediate, by-product, and product are depicted below (HPLC conditions A: Waters 2695 HPLC system using a C18 SunFire column 3.5 µm, 4.6 x 150 mm; UV detection at 250 nm; eluent 40:60 of 0.1 vol% aqueous 85% phosphoric acid/acetonitrile, respectively at flow rate of 1 mL/minute). By-product imide is formed as a result of acylation of product enamide in presence of acetic anhydride. However, it is hydrolyzed to the enamide with aqueous NaOH in presence of
tetra-n-butylammonium hydroxide.
13.
Alternatively, the progress of the reaction can also be monitored by thin layer chromatography (TLC) on Silicycle precoated 250-µm silica gel 60 F254 plates, elution with 40:60 ethyl acetate/hexanes, and visualization with UV light. The R
f values for the starting material, intermediate and product are given below with their structures.
14.
The reaction solution was heated at reflux overnight to ensure complete consumption of acylated oxime as determined by TLC (R
f 0.9)
(Note 13). By HPLC (conditions A, Note 12), 69.7 area% product, R
t 2.5 min; 21.5 area% imide, R
t 4.3 min .
15.
Tetra-n-butylammonium hydroxide as a 1 N solution in MeOH was purchased from Aldrich Chemical Company, Inc. and used as supplied.
16.
After 2 h, HPLC analysis (conditions A, see Note 12) showed 92.4 area% of enamide (R
t 2.5 min) and no imide (R
t 4.3 min).
17.
The color of the organic phase changes from dark brown to light yellow after the acid wash. The pH of the aqueous phase was <7 as determined by pH paper.
18.
Toluene can be used to adjust the slurry to ~80 g or 85 mL.
19.
Reagent grade
heptane was purchased from EMD Chemicals, Inc. and used as supplied.
20.
Up to 50 mL of the total
100 mL heptanes may be required to form a cloudy solution; however, in this case only 30 mL of heptanes was added.
21.
The reaction mixture was stirred overnight for convenience only. Stirring for 4-6 h at room temperature is typically sufficient.
22.
The product displays the following spectroscopic properties: Mp 138139 °C;
1H NMR
pdf (400 MHz, CDCl
3, mixture of rotamers) δ: 1.94 (s, 0.7 H), 2.14 (s, 2.3 H), 2.332.36 (m, 1.5 H), 2.372.41 (m, 0.5 H), 2.75 (t, 1.5 H,
J = 7.9 Hz), 2.83 (bt, 0.5 H,
J = 7.9 Hz), 5.95 (m, 0.25 H), 6.39 (t, 0.75 H,
J = 4.8 Hz), 6.83 (br s, 0.25 H), 7.00 (br s, 0.75 H), 7.127.22 (m, 4 H);
13C NMR
pdf (100 MHz, CDCl
3) δ: 20.3, 22.1, 22.6, 24.1, 27.2, 27.5, 119.6, 120.6, 121.9, 125.9, 126.3, 126.8, 127.4, 127.8, 128.1, 131.5, 136.7, 169.2; Anal. calcd. for C
12H
13NO: C, 76.56; H, 7.50; N, 7.44; found: C, 76.30; H, 7.37; N, 7.40.
23.
The purity of the product was determined to be >98 area% by HPLC analysis [R
t 2.5 min (conditions A), R
t 7.4 min (conditions B)
(Note 5)]. An analytical sample was prepared by dissolving 1 mg of sample in 5 mL of eluent and analyzed on a Waters 2695 HPLC system (conditions A) or Hewlett Packard 1100 HPLC system (conditions B)
(Note 5).
Handling and Disposal of Hazardous Chemicals
The procedures in this article are intended for use only by persons with prior training in experimental organic chemistry. All hazardous materials should be handled using the standard procedures for work with chemicals described in references such as "Prudent Practices in the Laboratory" (The National Academies Press, Washington, D.C., 2011 www.nap.edu). All chemical waste should be disposed of in accordance with local regulations. For general guidelines for the management of chemical waste, see Chapter 8 of Prudent Practices.
These procedures must be conducted at one's own risk. Organic Syntheses, Inc., its Editors, and its Board of Directors do not warrant or guarantee the safety of individuals using these procedures and hereby disclaim any liability for any injuries or damages claimed to have resulted from or related in any way to the procedures herein.
3. Discussion
Enantiomerically pure amines and their derivatives are valuable building blocks for organic synthesis and are an important class of biologically active compounds. In recent years, significant progress has been made to access chiral amines catalytically by asymmetric hydrogenation of enamides.
2 As a result, there has been an explosive growth in the development of chiral ligands for the catalytic hydrogenation of enamides. Despite the availability of a large number of catalysts for this transformation, the methods for the synthesis of enamides from the corresponding carbonyl compounds are still limited.
3 The procedure reported by Burk et al.
3a in 1998 is still the most frequently used method for the small scale preparation of enamides from ketones. Due to the difficulty with the scale-up of this procedure, a few alternatives have been reported involving palladium-catalyzed coupling of enol triflates or tosylates, derived from the corresponding ketones, with acetamide.
4 The procedure reported herein incorporates a similar approach as the Burk procedure, but is more scaleable and high-yielding, specifically for the synthesis of tetralone-based enamides.
5
Various phosphines were found to be effective for this transformation as depicted in Table 1. Due to the highly water-soluble nature of the triethylphosphine oxide by-product, the use of triethylphosphine was preferred, enabling easy isolation of enamide.
Table 1. Effect of Various Phosphines on Enamide Formationa
A variety of benzylic ketones and non-benzylic ketones were successfully converted to the corresponding enamides in reasonable yields (Tables 2 and 3). The substituted tetralone-based enamide (entry 4, Table 2) has been successfully scaled up to multi-kilogram scale.
Table 2. Enamides from Benzylic Ketones via Oximesa
Table 3. Enamides from Non-benzylic Ketones via Oximesa
Appendix
Chemical Abstracts Nomenclature (Collective Index Number);
(Registry Number)
3,4-Dihydronaphthalene-1(2H)-one oxime; (3349-64-2)
Hydroxylamine hydrochloride: (5470-11-1)
α-Tetralone: 1(2H)-Naphthalenone, 3,4-dihydro-; (529-34-0)
N-(3,4-Dihydronaphthalene-1-yl)acetamide: Acetamide, N-(3,4-dihydro-1-naphthalenyl)-; (213272-97-0)
Triethylphosphine; (554-70-1)
Acetic anhydride; (108-24-7)
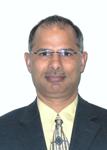 |
Surendra Singh obtained his Ph.D. in Organic Chemistry in India in 1988. He did postdoctoral work at Technion, Israel with Prof. Ehud Keinan, and at Ohio State University with Prof. Viresh Rawal working in the areas of asymmetric synthesis, radical chemistry and enzymatic reactions. In 1994, he joined Merck & Co in Technical Operations group as Senior Process Scientist. At Merck, he was involved in the development of chemical processes, which spans from laboratory development through production scale and their technical transfer to the manufacturing site. In 2001, he moved to Sepracor where he has been involved in the process research and development of NCEs and improved chemical entities. Currently, he is Director of Chemical Process Research. His research interests include the design of practical, environmentally benign, and economically viable processes for drug substances.
|
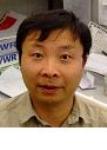 |
Hang Zhao was born in HangZhou, China and received his B.S. degree from Beijing University, China in 1985. In 1999 he completed his doctoral studies under the supervision of Prof. David Mootoo at the City University of New York. He then began postdoctoral research with Prof. Robert Holton at Florida State University working on the reconstruction and SAR studies on Taxol. His research interests include asymmetric synthesis and the development of new synthetic methods.
|
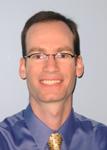 |
Charles P. Vandenbossche was born in 1971 in Detroit, MI. He received his B.A. in Chemistry (1994) and M.S. (1996) degrees from Wayne State University (Detroit), working under the supervision of Prof. James H. Rigby. Upon graduation, he relocated to Marlborough, MA, and joined the pharmaceutical company, Sepracor Inc. He is currently a Sr. Research Chemist in the Chemical and Pharmaceutical Sciences Department working as a process development chemist.
|
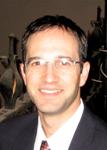 |
Stefan G. Koenig received his undergraduate degree in chemistry from Providence College in 1997. He completed his Ph.D. under Prof. David J. Austin at Yale University by developing a transient-linkage Pauson-Khand cyclization strategy to the marine natural product palau'amine. In 2002, he joined Prof. Andrea T. Vasella at the Swiss Federal Institute of Technology / ETH Zurich to develop a scalable process toward stable analogs of guanofosfocin, an important antifungal. He has been with Sepracor Process Research & Development since 2004, where his contributions have ranged from route scouting to API manufacture.
|
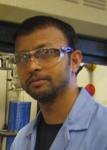 |
Gopal K. Datta was born in Calcutta, India. He graduated from Presidency College, the University of Calcutta with a Master of Science in Chemistry and specialized in organic chemistry. In 2008, Gopal K. Datta received his Ph.D. in Medicinal Chemistry from the division of Organic Pharmaceutical Chemistry, Department of Medicinal Chemistry, Faculty of Pharmacy, Uppsala University, Sweden under the supervision of Prof. Mats Larhed and Prof. Anders Hallberg. In 2009, Gopal K. Datta started his postdoctoral work in chemical biology at Prof. Jonathan A. Ellman's laboratory in the Department of Chemistry, University of California at Berkeley.
|
Copyright © 1921-, Organic Syntheses, Inc. All Rights Reserved