Org. Synth. 2010, 87, 330
DOI: 10.15227/orgsyn.087.0330
NICKEL-CATALYZED ENANTIOSELECTIVE NEGISHI CROSS-COUPLINGS OF RACEMIC SECONDARY α-BROMO AMIDES WITH ALKYLZINC REAGENTS: (S)-N-BENZYL-7-CYANO-2-ETHYL-N-PHENYLHEPTANAMIDE
[Heptanamide, 7-cyano-2-ethyl-N-phenyl-N-(phenylmethyl)-, (2S)-]
Submitted by Sha Lou and Gregory C. Fu
1.
Checked by Christopher S. Bryan and Mark Lautens.
1. Procedure
A. (5-Cyanopentyl)zinc(II) bromide (1). An oven-dried, 200-mL pear-shaped Schlenk flask equipped with a magnetic stirbar (egg shaped, 25.4 × 12.7 mm) and an argon line connected to the standard taper outer joint is purged with argon for 5 min. Zinc powder (9.80 g, 150 mmol, 1.50 equiv) (Note 1) is added through the open neck, and then the flask is capped with a rubber septum and heated in an oil bath under high vacuum (0.5 mmHg) at 70 °C for 30 min. Then, the flask is refilled with argon, and anhydrous 1,3-dimethyl-2-imidazolidinone (DMI; 100 mL) (Note 2) is added via syringe. Iodine (I2) (634 mg, 2.50 mmol, 0.0250 equiv) (Note 3) is added in one portion through the neck. The neck is re-capped with a rubber septum, and the reaction mixture is stirred at 70 °C in an oil bath until the red color fades (~5 min). 6-Bromohexanenitrile (13.2 mL, 100 mmol, 1.00 equiv) (Note 4) is added via syringe over 4 min, and the reaction mixture is stirred at 70 °C for 12 h. Then, the oil bath is removed, and the mixture is allowed to cool at rt for 1 h without stirring. During this time, the unreacted zinc powder settles at the bottom of the flask. The flask is equipped with a fritted filter tube of medium porosity capped with an oven-dried, 2-necked 250-mL round-bottom flask, and the supernatant solution is filtered under argon by inverting the set-up (Note 5). The resulting clear yellow solution is employed in the next step without further purification. 1H NMR spectroscopy is used to determine that the concentration of the alkylzinc solution is 0.72 M (Note 6). This organozinc solution can be stored under argon at 0-4 °C for up to 3 weeks without deterioration.
B. (S)-N-Benzyl-7-cyano-2-ethyl-N-phenylheptanamide (2). An oven-dried, 1000-mL, three-necked, round-bottomed flask equipped with a thermometer inlet, thermometer, magnetic stirbar (egg shaped, 41.3 × 19.0 mm), and argon inlet is purged with argon for 20 min. NiCl2glyme (536 mg, 2.44 mmol, 0.0697 equiv) (Note 7), (R)-(i-Pr)-Pybox (960 mg, 3.19 mmol, 0.0910 equiv) (Note 8), and N-benzyl-2-bromo-N-phenylbutanamide (11.6 g, 35.0 mmol, 1.00 equiv) (Note 9) are then added through the open neck under a positive pressure of argon. The open neck is capped with a rubber septum, and DMI (72
mL) (Note 2) and THF (17.5 mL) (Note 10) are each added via syringe. The resulting orange solution is stirred at rt for 10 min, and then the organozinc reagent (0.72 M in DMI; 63.2 mL, 45.5 mmol, 1.30 equiv) is added via syringe over 6 min, maintaining the internal temperature below 30 °C. The resulting dark-brown reaction mixture is stirred at rt (temperature of the solution: 23 °C) for 24 h. The progress of the reaction can be monitored by 1H NMR analysis of an aliquot of the reaction mixture. After the α-bromo amide starting material is completely consumed (determined by observing the disappearance of a triplet at δ 3.95), the excess organozinc reagent is quenched by adding ethanol (15
mL). The brown reaction mixture is diluted with Et2O (500
mL), and the resulting solution is transferred to a 1-L separatory funnel and washed with H2O (300 mL × 3). The organic layer is dried over anhydrous Na2SO4 (30 g) and filtered through a Büchner funnel containing a bed of celite (1.0 cm height). The filtrate is concentrated by rotary evaporation (40 °C, 20 mmHg), and the resulting orange oil is purified by column chromatography on SiO2 (wet packed in hexanes; 8 cm diameter × 30 cm height; 350 g; eluting with a gradient of EtOAc in hexanes (500 mL of 10% EtOAc/hexanes, 1.5 L of 15% EtOAc/hexanes, 1.5 L of 20% EtOAc/hexanes, 1.0 L of 30% EtOAc/hexanes, 1.0 L of 40% EtOAc/ hexanes; 100-mL fractions) (Note 11). The cross-coupling product 2 has an Rf= 0.5 (TLC analysis on SiO2 (30% EtOAc/hexanes, visualization with a UV lamp) (Note 12). The desired product is obtained as a white solid (10.4-10.8 g, 85-89% yield, 91% ee) (Note 13).
2. Notes
1.
Zinc powder (99.9%, ~100 mesh) was purchased from Alfa Aesar and used as received.
2.
1,3-Dimethyl-2-imidazolidinone (≥ 99.5%, over molecular sieves, water content: =0.04%) was purchased from Aldrich and used as received.
3.
I2(≥99%, chips) was purchased from Aldrich and used as received.
4.
6-Bromohexanenitrile (95%) was purchased from Aldrich and used as received.
5.
The submitters described an alternative filtration procedure as depicted in Figure 1.
Figure 1.
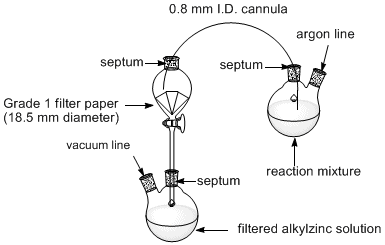
6.
For the NMR experiment, a sealed tube of D
2O was placed in an NMR tube containing the alkylzinc solution, and a standard 1D
1H NMR
pdf spectrum was obtained using D
2O as a reference. The resonances for DMI appear at δ 3.31 and 2.74. The resonance for the CH
2Zn protons of RZnBr appears at δ 0.13. The resonance for the terminal Me group of the reduction product appears at δ 0.94. The molar ratio of DMI : RZnBr : RH (X : 1 : Y) was determined by No-D NMR to be 11.7 : 1 : 0.088. The density (d) of the solution was measured to be 1.14 g/mL. The final concentration of alkylzinc was calculated to be 0.72 M according to the equation: [RZnBr] = d × 1000/(X × MW
DMI + MW
RZnBr + Y × MW
RH). The submitters measured the concentration using No-D NMR spectroscopy. For the No-D NMR experiment, a
1H NMR spectrum of a blank CDCl
3 sample was taken to lock the sample and determine the reference. Then, an unlocked No-D NMR spectrum of the alkylzinc sample in DMI was taken (pw = 1.5). The resonances for DMI appear at δ 4.08 and 3.51. The resonance for the CH
2Zn protons of RZnBr appears at δ 0.89. The resonance for the terminal Me group of the reduction product appears at δ 1.71. The molar ratio of DMI : RZnBr : RH (X : 1 : Y) was determined by No-D NMR to be 11.2 : 1 : 0.13. The density (d) of the solution was measured to be 1.20 g/mL. The final concentration of alkylzinc was calculated to be 0.78 M according to the equation: [RZnBr] = d × 1000/(X × MW
DMI + MW
RZnBr + Y × MW
RH).
7.
NiCl
2glyme was purchased from Strem and used as received.
8.
(
R)-(
i-Pr)-Pybox (99%) was purchased from Aldrich and used as received.
9.
N-Benzyl-2-bromo-N-phenylbutanamide (97%) may be purchased from Aldrich.
10.
THF (99+%) was purchased from J.T. Baker (water content: 24 ppm) and purified by passage through an activated alumina column under argon.
11.
Column chromatography was performed on SiO
2 (0.040-0.063 mm, pore diameter 6 nm purchased from Silicycle). The submitters report separation with smaller volumes of eluent (500 mL of 10% EtOAc/hexanes, 1.0 L of 15% EtOAc/hexanes, 1.0 L of 20% EtOAc/hexanes, 1.0 L of 30% EtOAc/hexanes, 500 mL of 40% EtOAc/hexanes) using an equal amount of Sorbent Technologies 60 Å silica gel.
12.
Analytical thin-layer chromatography was performed using EMD 0.25 mm silica gel 60-F plates.
13.
Compound
2 has the following physical properties: Mp: 67-69 °C; [α]
26D −30.5 (
c 1.05, CHCl
3);
1H NMR
pdf (CDCl
3, 500 MHz) δ;: 0.80 (t,
J = 7.4 Hz, 3 H), 1.11-1.28 (m, 2 H), 1.30-1.42 (m, 4 H), 1.56-1.69 (m, 4 H), 2.13-2.20 (m, 1 H), 2.27 (t,
J = 7.1 Hz, 2 H), 4.86 (d,
J = 14.0 Hz, 1 H), 4.95 (d,
J = 14.0 Hz, 1 H), 6.90-6.93 (m, 2 H), 7.18-7.20 (m, 2 H), 7.22-7.28 (m, 3 H), 7.30-7.35 (m, 3 H);
13C NMR
pdf (CDCl
3, 100 MHz) δ: 12.3, 17.2, 25.3, 26.4, 26.8, 28.9, 32.7, 43.7, 53.2, 120.0, 127.6, 128.1, 128.5, 129.2, 129.6, 138.0, 142.5, 175.9; IR (film): 3061, 2943, 2861, 2239, 1640, 1594, 1497, 1453, 1408, 1262, 1246, 1211, 1079, 1014, 782, 738, 701 cm
-1; MS (ESI)
m/z (rel. intensity) 387.2 (4), 372.2 (8), 371.2 (30), 350.2 (22), 349.2 (100); HRMS (ESI) calcd for C
23H
29N
2O ([M+H]
+) 349.2274, found, 349.2285; Anal. calcd. for C
23H
28N
2O: C, 79.27; H, 8.10; N, 8.04; found: C, 79.23; H, 8.40; N, 8.10. The spectral data are in agreement with reported values.
2 The ee was determined by HPLC analysis with an Agilent 1100 Series HPLC system equipped with a CHIRALPAK AD-H column (length 250 mm, I.D. 4.6 mm) (hexanes/isopropanol 93:7, 1.0 mL/min) with t
r (major) = 20.2 min, t
r (minor) = 21.7 min. The peak areas were measured at λ = 210 nm. The ee can also be determined by supercritical fluid chromatography (SFC) analysis on a Berger SFC MiniGram system: CHIRALPAK AD-H column (length 250 mm, I.D. 4.6 mm); solvent system: 15% MeOH, 3.0 mL/min; retention times: t
r (major) = 2.39 min, t
r (minor) = 2.57 min. The purity of compound
2 (98%) was determined by HPLC analysis (t
r = 6.30 min) with an Agilent 1100 Series HPLC system equipped with an Eclipse XDB-C18 column (length 150 mm, I.D. 4.6 mm, particle size 5 μm), using 98% MeOH/(0.2% AcOH in water) for 5 min, with a flow rate of 0.8 mL/min. Peak areas were measured at λ = 210 nm.
Safety and Waste Disposal Information All hazardous materials should be handled and disposed of in accordance with "Prudent Practices in the Laboratory"; National Academy Press; Washington, DC, 1995.
3. Discussion
Substantial advances have recently been described in the development of catalysts that cross-couple alkyl electrophiles.
3 For couplings of unsymmetrical secondary electrophiles, there is the potential to control the stereochemistry at the carbon that bears the leaving group.
4 This stereochemical aspect adds an important dimension to carbon-carbon bond-forming reactions of alkyl electrophiles. In 2003, we reported that Ni(cod)
2/(
s-Bu)-Pybox catalyzes Negishi reactions of secondary alkyl bromides and iodides.
5 Our observation that a chiral Pybox is the ligand of choice raised the obvious question of whether it might be possible to develop a highly enantioselective alkyl-alkyl cross-coupling.
We have determined that this objective can be achieved with certain electrophiles, including α-bromo amides.
6,7 Thus, NiCl
2glyme/(
i-Pr)-Pybox catalyzes the cross-coupling of a racemic mixture of an α-bromo amide with an array of alkylzinc reagents
8 in good ee and yield (Table 1). The catalyst tolerates a variety of functional groups, such as olefins, ethers, imides, and nitriles.
Table 1. Enantioselective, Stereoconvergent Negishi Cross-Couplings of α-Bromo Amides with Alkylzinc Reagents.
In this stereoconvergent process, both enantiomers of the racemic substrate are transformed into the same enantiomer of the product with good stereoselectivity. There is no evidence for kinetic resolution of the starting material during the catalytic asymmetric Negishi reaction. The cross-coupling product can be converted into other useful families of compounds, such as primary alcohols (reduction with LiAlH4).
Appendix
Chemical Abstracts Nomenclature (Collective Index Number);
(Registry Number)
6-Bromohexanenitrile: Hexanenitrile, 6-bromo-; (6621-59-6)
Zinc; (7440-66-6)
N-Benzyl-2-bromo-N-phenylbutanamide: Butanamide, 2-bromo-N-phenyl-N-(phenylmethyl)-; (851073-30-8)
Nickel(II) chloride, dimethoxyethane adduct (NiCl2glyme): Nickel, dichloro[1,2-di(methoxy-κO)ethane]-; (29046-78-4)
(R)-i-Pr-Pybox: Pyridine, 2,6-bis[(4R)-4,5-dihydro-4-(1-methylethyl)-2- oxazolyl]-; (131864-67-0)
(S)-N-Benzyl-7-cyano-2-ethyl-N-phenylheptanamide: Heptanamide, 7-cyano-2-ethyl-N-phenyl-N-(phenylmethyl)-, (2S)-; (851 073-44-4)
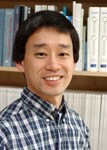 |
Prof. Greg Fu was born in Galion, Ohio, in 1963. He received a B.S. degree in 1985 from MIT, where he worked in the laboratory of Prof. K. Barry Sharpless. After earning a Ph.D. from Harvard in 1991 under the guidance of Prof. David A. Evans, he spent two years as a postdoctoral fellow with Prof. Robert H. Grubbs at Caltech. In 1993, he returned to MIT, where he is currently the Firmenich Professor of Chemistry. Prof. Fu received the Springer Award in Organometallic Chemistry in 2001, the Corey Award of the American Chemical Society in 2004, and the Mukaiyama Award of the Society of Synthetic Organic Chemistry of Japan in 2006. He is a fellow of the Royal Society of Chemistry and of the American Academy of Arts and Sciences. Prof. Fu serves as an associate editor of the Journal of the American Chemical Society.His current research interests include metal-catalyzed coupling reactions, chiral-ligand design, and enantioselective nucleophilic catalysis. |
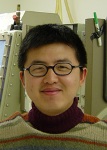 |
Sha Lou was born in He Bei, China, in 1979. He received a B.S. in Chemistry from Beijing University of Chemical Technology in 2002, where he conducted undergraduate research on fullerene functionalizations with Professor Shenyi Yu. He obtained a Ph.D. degree in January 2008 from Boston University under the direction of Professor Scott E. Schaus. His graduate research focused on transition metal- and organic molecule-catalyzed asymmetric carbon-carbon bond-forming reactions and synthesis. In 2008, he joined the group of Professor Greg Fu at MIT as a postdoctoral fellow. His current research involves the development of transition metal-catalyzed enantioselective cross-coupling reactions. |
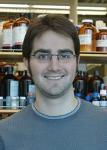 |
Christopher Bryan was born in Winnipeg, Canada in 1982. He received his B.Sc. degree with distinction in 2005 from the University of Victoria, where he worked in the laboratory of Dr. Scott McIndoe. While an undergraduate, he worked as a Co-op student in the medicinal chemistry department at Boehringer-Ingelheim Pharmaceuticals in Laval, PQ. He is currently pursuing his Ph. D. at the University of Toronto under the supervision of Professor Mark Lautens. His research is focused on the synthesis of heterocycles via metal-catalyzed tandem processes. |
Copyright © 1921-, Organic Syntheses, Inc. All Rights Reserved