Org. Synth. 2011, 88, 14-21
DOI: 10.15227/orgsyn.088.0014
THE PREPARATION OF AMIDES BY COPPER-MEDIATED OXIDATIVE COUPLING OF ALDEHYDES AND AMINE HYDROCHLORIDE SALTS
Submitted by Maxime Giguère-Bisson, Woo-Jin Yoo, and Chao-Jun Li
1.
Checked by Melissa J. Leyva and Jonathan A. Ellman.
1. Procedure
Caution! Reactions and subsequent operations involving peracids and peroxy compounds should be run behind a safety shield. Peroxy compounds should be added to the organic material, never the reverse. For relatively fast reactions, the rate of addition of the peroxy compound should be slow enough so that it reacts rapidly and no significant unreacted excess is allowed to build up. The reaction mixture should be stirred efficiently while the peroxy compound is being added, and cooling should generally be provided since many reactions of peroxy compounds are exothermic. New or unfamiliar reactions, particularly those run at elevated temperatures, should be run first on a small scale. Reaction products should never be recovered from the final reaction mixture by distillation until all residual active oxygen compounds (including unreacted peroxy compounds) have been destroyed. Decomposition of active oxygen compounds may be accomplished by the procedure described in Korach, M.; Nielsen, D. R.; Rideout, W. H. Org. Synth. 1962, 42, 50 (Org. Synth. 1973, Coll. Vol. 5, 414). [Note added April 2018].
All the following manipulations are performed in air.
A mechanical stir paddle (60 mm in diameter) is fitted to an oven-dried 100-mL, 2-necked round-bottomed flask (24/40 joint). Copper (I) iodide (0.047 g, 0.25 mmol, 0.01 equiv) (Note 1), AgIO3 (0.070 g, 0.25 mmol, 0.01 equiv) (Note 2), L-valine methyl ester hydrochloride (6.19 g, 36.9 mmol, 1.5 equiv) (Note 3) and CaCO3 (2.10 g, 21.0 mmol, 0.9 equiv) (Note 4) are added sequentially to the reaction flask, and the mixture is stirred at the maximum rate (the submitters stirred at 230 rpm). Acetonitrile (5.5 mL) (Note 5) is added in one portion using a syringe under constant stirring. A white opaque mixture is obtained at the end of this step. Benzaldehyde (2.50 mL, 24.6 mmol, 1.0 equiv) (Note 6) is added over 15 seconds using a syringe under constant stirring, and upon addition the reaction mixture becomes an opaque yellow. A tert-butyl hydroperoxide solution (4.00 mL, 28.0 mmol, 1.1 equiv) (Note 7) is added using a syringe under constant stirring and the mixture turns green. The reaction flask is then placed in an oil bath at 40 °C for 6 h under constant stirring.
After placing the reaction flask in the oil bath, the reaction mixture gradually becomes a clear orange-gold solution. The reaction flask is taken out of the oil bath and is placed in an ice-bath. Upon cooling, the reaction mixture becomes a thick, opaque mustard-yellow. Hydrochloric acid (1.6 M, 7.5 mL) is added dropwise (ca. 4 drops per second) under constant stirring. Upon neutralization, the reaction mixture turns green. The reaction flask is taken out of the ice-bath and ethyl acetate (25 mL) (Note 8) and distilled water (20 mL) are added. The organic layer is light green and the aqueous layer white-blue and opaque. If left overnight at room temperature, the organic layer may turn orange. The mixture is transferred to a 250-mL separatory funnel. The 100-mL round-bottomed flask is rinsed with ethyl acetate (15 mL) and distilled water (15 mL). The rinsings are transferred to the separatory funnel. Brine (15 mL) is added to the separatory funnel to obtain a better phase separation. The two layers are separated and transferred into 250-mL Erlenmeyer flasks. The aqueous layer is then transferred back to the separatory funnel and extracted with ethyl acetate (3 × 25 mL). Brine (3 × 15 mL) is added to facilitate every extraction. The organic layers are combined and washed with a saturated solution of NaHCO3 (25 mL) and poured into a 250-mL Erlenmeyer flask. Anhydrous magnesium sulfate (4.0 g) (Note 9) is added. The solution is filtered under vacuum using a 30-mL suction funnel equipped with a medium porosity fritted-disc into an oven-dried, 500-mL round-bottomed flask. The magnesium sulfate in the frit is washed with two portions of ethyl acetate (2 × 10 mL), and the combined filtrate is concentrated under vacuum (40 °C and 21 mmHg) to provide a white-beige solid. Dichloromethane (20 mL) (Note 10) is added to the round-bottomed flask. The flask is sonicated for 30 seconds resulting in a green brown solution. Silica gel (5.0 g) (Note 11) is added to the flask and the solvent is evaporated using a rotary evaporator at 55-60 °C (21 mmHg). Flash column chromatography is performed using a 5-cm-wide, 45-cm-high column packed with 230 g of silica gel. The column is packed with silica gel, flushed with 1000 mL of the eluent (hexanes: ethyl acetate = 3:2) (Note 12). The eluent level is adjusted to the upper level of the silica gel. The silica gel containing the compound is then loaded on the column and column chromatography is then performed (1.7 L of eluent is used). The collected fractions (15 mL each) are analysed using TLC (eluting with hexanes: ethyl acetate =3:2) and the spots are visualized using a UV lamp (254 nm) (Note 13). The fractions (41 to 92) containing the desired compound are combined and evaporated to dryness using a rotary evaporator. The product is further dried under reduced pressure (0.01 mmHg) for 2 h to give (S)-methyl-2-benzamido-3-methylbutanoate (4.91 g, 20.9 mmol, 85% yield) (Note 14).
2. Notes
1.
Copper (I) iodide was obtained from Aldrich and used as received.
2.
Silver iodate was obtained from GFS Chemicals and used as received.
3.
L-Valine methyl ester hydrochloride 99% was obtained from Aldrich and used as received.
4.
Calcium carbonate A.C.S reagent was obtained from Aldrich and used as received.
5.
Acetonitrile A.C.S. Grade was obtained from Fisher Scientific and used as received.
6.
Benzaldehyde ReagentPlus ≥99% was obtained from Aldrich and used as received.
7.
tert-Butyl hydroperoxide, T-hydro solution, 70 wt. % in water was obtained from Aldrich and used as received.
8.
Ethyl acetate HPLC Grade was obtained from Fisher Chemicals and used as received.
9.
Anhydrous
magnesium sulfate was obtained from Fisher Chemicals and used as received.
10.
Dichloromethane A.C.S. Reagent was obtained from ACP Chemicals and used as received.
11.
Silica gel MP Silitech 32-63 D 60 Å was used as received.
12.
Hexanes HPLC Grade was obtained from Fisher Chemicals and used as received.
13.
The reaction and column fractions were monitored by TLC using Dynamic Adsorbents, Inc. glass plates coated with 250 mm F-254 silica gel:
Hexanes:ethyl acetate (3:2). The benzaldehyde starting material has an R
f of 0.65, and the product
(S)-methyl-2-benzamido-3-methylbutanoate has an R
f of 0.45.
14.
The product is obtained as a white (slightly yellow) flaky solid and has the following physical and spectroscopic properties: Melting point: 108-109 °C, IR (neat): 3347, 2967, 1736, 1640, 1518, 1490, 1202, 1151, 994, 692 cm
−1.
1H NMR
pdf (400 MHz, CDCl
3) δ: 0.96 (d,
J = 7.0 Hz, 3 H), 0.98 (d,
J= 7.0 Hz, 3 H), 2.25 (m, 1 H), 3.74 (s, 3 H), 4.74 (dd,
J= 5.2, 8.4 Hz, 1 H), 6.64 (d,
J= 8.4 Hz, 1 H), 7.39 (m, 2 H), 7.48 (m, 1 H), 7.77 (m, 2 H).
13C NMR
pdf (100 MHz, CDCl
3) δ: 18.1, 19.2, 31.8, 52.4, 57.6, 127.2, 128.8, 131.9, 134.3, 167.5, 172.9. MS (ESI) m/z [M + H]
+ calcd for
C13H18NO3: 236.128. Found: 236.128. HPLC (
Daicel Chiral AD-H, hexanes/isopropanol=95:5, flow rate 1.0 mL/min) t
R = 20.556 min (major), < 99.9 % ee. Submitters provided checkers with racemic product, t
R = 15.150 min (minor). Anal. calcd. for
C13H17NO3: C, 66.36; H, 7.28; N, 5.95. Found: C, 66.38; H, 7.44, N, 5.85. Specific rotation, [α]
D20 +34.0
° (c = 0.1, CH
2Cl
2)
Handling and Disposal of Hazardous Chemicals
The procedures in this article are intended for use only by persons with prior training in experimental organic chemistry. All hazardous materials should be handled using the standard procedures for work with chemicals described in references such as "Prudent Practices in the Laboratory" (The National Academies Press, Washington, D.C., 2011 www.nap.edu). All chemical waste should be disposed of in accordance with local regulations. For general guidelines for the management of chemical waste, see Chapter 8 of Prudent Practices.
These procedures must be conducted at one's own risk. Organic Syntheses, Inc., its Editors, and its Board of Directors do not warrant or guarantee the safety of individuals using these procedures and hereby disclaim any liability for any injuries or damages claimed to have resulted from or related in any way to the procedures herein.
3. Discussion
The amide functional group is ubiquitous in organic chemistry and is an important motif in polymers, natural products, and pharmaceuticals.
2 The most prevalent strategy for amide bond formation relies heavily upon the interconversion of activated
carboxylic acid derivatives with an amine.
3 However, due to the lability of activated
carboxylic acid derivatives, alternative strategies toward the synthesis of amides have been explored. Examples include the utilization of azides as amine equivalents in the modified Staudinger reaction,
4 hydrative amide syntheses with alkynes,
5 and
thio acid/ester ligation methods.
6 Transition-metal-catalyzed carbonylation of alkenes,
7 alkynes,
8 and haloarenes
9 with amines has also been employed for amide synthesis. Finally, the direct coupling of aldehydes with amines under oxidative conditions can also serve as an attractive entry into amides.
10 This methodology has been hampered thus far by the need for expensive transition-metal catalysts and poor substrate scope. We previously reported a copper-catalyzed stereoselective oxidative esterification of aldehydes with β-dicarbonyl compounds using
tert-butyl hydroperoxide (TBHP) as an oxidant.
11 In light of our success in that oxidative esterification reaction, we turned our attention to the much more challenging amidation reaction of simple aldehydes and amines. Subsequently, we developed a simple methodology
12 using CuI as catalyst, an aqueous solution of
tert-butyl hydroperoxide as an oxidant, as well as
AgIO3 as an additive. This paper describes the scale up of this methodology. The specific reaction shown in this paper is the oxidative amidation of
benzaldehyde with the
hydrochloride salt of
L-valine methyl ester. The
CuI/TBHP/AgIO3 methodology has also been used successfully on a smaller scale with other aldehydes and amine hydrochloride salts as shown in the following table.
Table 1. Copper Catalyzed Oxidative Amidation of Aldehydes with Amine Hydrochloride Saltsa,b
Appendix
Chemical Abstracts Nomenclature (Collective Index Number);
(Registry Number)
L-Valine methyl ester hydrochloride: (S)-2-amino-3-methylbutanoate hydrochloride; (6306-52-1)
Silver iodate: iodic acid (HIO3), silver(1+) salt (1:1); (7783-97-3)
Calcium carbonate: carbonic acid, calcium salt (1:1); (471-34-1)
tert-Butyl hydroperoxide: 1,1-dimethylethyl hydroperoxide; (75-91-2)
Benzaldehyde: benzenecarboxaldehyde; (100-52-7)
(S)-Methyl-2-benzamido-3-methylbutanoate: L-Valine, N-benzoyl-, methyl ester; (1
0512-91-1)
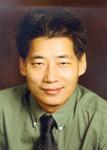 |
Chao-Jun Li (born in 1963) received his Ph.D at McGill University (1992). After a two year NSERC Postdoctoral position at Stanford University, he became Assistant Professor (1994), Associate Professor (1998) and Full Professor (2000) at Tulane University. In 2003, he became a Canada Research Chair (Tier I) in Organic/Green Chemistry and a Professor (E. B. Eddy Chair Professor since 2010) of Chemistry at McGill University in Canada. Currently, he serves as the Co-Chair of the Canadian Green Chemistry and Engineering Network, Director of CFI Facility for Green Chemistry and Green Chemicals, and Co-Director for FQRNT Center for Green Chemistry and Catalysis. His current research efforts are focused on developing innovative and fundamentally new organic reactions that will defy conventional reactivities and have high synthetic efficiency. |
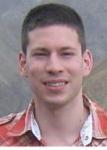 |
Maxime Giguère-Bisson was born in 1986 in Grand-Mère (Canada). He obtained his B. Sc. Degree in Chemistry Honours in 2008 from McGill University. He is currently doing his Masters in Chemistry under the supervision of Dr. Chao-Jun Li. His current research focuses on Asymmetric A3-Coupling. |
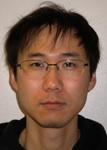 |
Woo-Jin Yoo (born in 1978) received his B.Sc. degree from the University of Guelph in 2003. In 2005, he received his M.Sc. degree from the University of Guelph under the supervision of William Tam, where he studied metal-catalyzed cross-coupling reactions and Diels-Alder cycloadditions. He then joined the research group of Chao-Jun Li at McGill University and studied copper-catalyzed oxidative coupling reactions and multicomponent coupling reactions. He obtained his Ph.D. degree in 2009 and is currently a postdoctoral fellow with Shu Kobayashi at The University of Tokyo. He has been the recipient of the Alexander Graham Bell Canada Graduate Scholarship (Ph.D.), an NSERC Postdoctoral Fellowship (declined), and a JSPS Postdoctoral Fellowship for Foreign Researchers. |
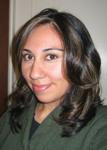 |
Melissa J. Leyva was born in El Paso, Texas in 1982. She received her B.S. degree in Chemistry at the University of Texas, El Paso in 2005. She then began her doctoral studies at the University of California, Berkeley under the direction of Professor Jonathan A. Ellman. Her graduate research has focused on the identification of novel inhibitors for therapeutically important proteases. |
Copyright © 1921-, Organic Syntheses, Inc. All Rights Reserved