Org. Synth. 2011, 88, 138-151
DOI: 10.15227/orgsyn.088.0138
PHOSPHINE-CATALYZED [3 + 2] ANNULATION: SYNTHESIS OF ETHYL 5-(tert-BUTYL)-2-PHENYL-1-TOSYL-3-PYRROLINE-3-CARBOXYLATE
Submitted by Ian P. Andrews and Ohyun Kwon
1
.
Checked by John T. Colyer, Oliver R. Thiel, and Margaret Faul.
1. Procedure
A.
Ethyl 5,5-dimethylhexa-2,3-dienoate (
1
).
A flame-dried 2-L 3-necked, round-bottomed flask equipped with an overhead mechanical stirrer (Teflon paddle, 6 × 2 × 0.3 cm), a temperature probe, and a nitrogen inlet is charged with
ethyl (triphenylphosphoranylidene)acetate (46.4 g, 133 mmol, 1.00 equiv)
(Note 1),
dichloromethane (665 mL)
(Note 9), and
triethylamine (20.4 mL, 146 mmol, 1.10 equiv)
(Note 10). The mixture is stirred under nitrogen until a homogeneous solution is achieved.
3,3-Dimethylbutyryl chloride (18.5 mL, 133 mmol, 1.00 equiv)
(Note 11) is added over 1 h using an addition funnel. The reaction mixture is stirred at room temperature for an additional 18 h during which time a precipitate forms, resulting in a cloudy heterogeneous mixture. The reaction mixture is added in three portions to a 1-L round-bottomed flask and concentrated to a volume of ca. 230 mL using a rotary evaporator (20 °C, 20-25 mmHg).
Hexanes (300 mL)
(Note 12) is added, and the flask is manually swirled for 2 min, resulting in a precipitate. The heterogeneous suspension is filtered through silica gel (100 g) (Note 13) in a 600-mL glass-fritted medium porosity Büchner funnel into a 2-L round-bottomed flask. The filter cake is rinsed with
hexanes (600 mL). The resulting slightly yellow solution is concentrated under rotary evaporation (20 °C, 20-25 mmHg) until 750 mL of the solvent has been removed (Note 14). The heterogeneous mixture is filtered through silica gel (100 g) (Note 13) in a 600-mL glass fritted medium porosity Büchner funnel into a 2-L round-bottomed flask. The filter cake is rinsed with 500 mL of
hexanes/diethyl ether
(19:1) (Note 15). The solution is concentrated (20 °C, 20-25 mmHg) and left on vacuum overnight to give
ethyl 5,5-dimethylhexa-2,3-dienoate (1) (16.4-16.6 g, 73-74%) (Notes 16 and 17) as a pale yellow oil.
B.
Ethyl 5-(tert-butyl)-2-phenyl-1-tosyl-3-pyrroline-3-carboxylate (
2
).
A flame-dried, 1-L 3-necked, round-bottomed flask, equipped with an overhead mechanical stirrer (Teflon paddle, 6 × 2 × 0.3 cm), temperature probe, and a nitrogen inlet, is charged with
(E)-N-benzylidene-4-methylbenzenesulfonamide (7.80 g, 30.0 mmol, 1.00 equiv)
(Note 18) and purged with argon.
Benzene (300 mL)
(Note 19) is added to the flask via syringe, and the mixture is stirred until a homogeneous solution is achieved. Tributylphosphine (1.5 mL, 6.0 mmol, 0.20 equiv) (Note 20) is added via syringe and the reaction mixture is stirred for 5 min.
Ethyl 5,5-dimethylhexa-2,3-dienoate (1) (6.06 g, 36.0 mmol, 1.20 equiv) is added over 5 min using a syringe pump (Note 21). The reaction is monitored using TLC (Note 22). Upon completion, the mixture is transferred into a 1-L round-bottomed flask and concentrated under rotary evaporation (30 °C, 20-25 mmHg) to a thick orange/brown oil and placed under high vacuum for 10 min (0.1-0.2 mmHg) to ensure the removal of residual solvent (Note 23). A sample of the crude oil (est. 0.5 g) is purified by column chromatography to afford seed crystals of 2 (Notes 24 and 25). A mixture of
hexanes/ethyl acetate (9:1, 75 mL) is added to the crude oil in a 1-L round-bottomed flask and a reflux condenser is attached. A Teflon-coated stir bar is added and the crude residue is dissolved by heating in an oil bath with stirring until a homogeneous solution is achieved. After cooling to room temperature, the reflux condenser is removed and the flask is capped with a plastic stopper and left to stand overnight. The seed crystals are added as the reaction mixture is cooled. The following day the mixture is placed in a freezer at -20 °C for 2 h. The crystals that precipitated are collected under vacuum filtration using a glass-fritted funnel (Notes 26 and 27). The crystals are rinsed with
hexanes (200 mL) to give 11.4-11.8 g (88-92% yield) of 2 as a white to pale yellow solid (Note 28).
2. Notes
1.
Ethyl (triphenylphosphoranylidene)acetate
is commercially available from Aldrich or can be prepared readily from ethyl bromoacetate
(Note 2) and triphenylphosphine
(Note 3) using the following procedure provided by the submitters: A 1-L round-bottom flask equipped with a magnetic stir bar is charged with triphenylphosphine (39.4 g, 150 mmol) and
toluene (200 mL)
(Note 4). The mixture is stirred until a homogeneous solution is achieved.
Ethyl bromoacetate (16.6 mL, 150 mmol) is added via syringe. The mixture is capped with a plastic stopper and stirred at room temperature for 24 h, during which time a white precipitate is formed, resulting in a thick slurry. The precipitate is collected under vacuum filtration using a glass-fritted Büchner funnel. The filtrate is washed with 75 mL of toluene
(Note 5) and 75 mL of diethyl ether
(Note 6). The white solid is transferred to a 1-L round-bottomed flask, placed under vacuum for 2 h, and then dissolved in 450 mL of deionized water
(Note 7). A magnetic stir bar is added, followed by phenolphthalein indicator solution (200 μL)
(Note 8). While stirring, 2 M potassium hydroxide is added dropwise until a pink color persists. The aqueous layer is extracted with
dichloromethane (3 × 200 mL)
(Note 9) and the combined organic extracts are washed with
brine (400 mL), dried over sodium sulfate, filtered, and concentrated under rotary evaporation (30 °C, 20-25 mmHg). The resulting thick oil is redissolved in 20 mL of
diethyl ether
(Note 6) and concentrated under rotary evaporation (30 °C, 20-25 mmHg) to give an off-white solid. Drying under high vacuum (0.1-0.2 mmHg) yields 48.1 g (92%) of
ethyl (triphenylphosphoranylidene)acetate
as an off-white solid, mp 118-121 °C. The checkers used commercially available material from Aldrich (95%).
2.
Ethyl bromoacetate (98%) was purchased from Alfa Aesar and used as received.
3.
Triphenylphosphine (99%) was purchased from Alfa Aesar and used as received.
4.
Toluene (99.8%) was purchased from Fisher Scientific and used as received.
5.
Some of the precipitate may stick to the walls of the flask. If so, toluene can be used to rinse the round-bottomed flask.
6.
Diethyl ether (99.9%) was purchased from Fisher Scientific and used without further purification.
7.
If the addition of water does not result in a homogeneous solution, heating is applied to aid the solubilization of the phosphonium salt.
8.
Phenolphthalein indicator solution is prepared by dissolving phenolphthalein powder (100 mg) (purchased from Mallinckrodt and used as received) in
2-propanol (10 mL) (99.9% purchased from Fisher Scientific and used as received). The addition of this indicator is used to help avoid saponification of the ethyl ester, which occurs upon the addition of excess potassium hydroxide.
9.
Dichloromethane (99.9%) as purchased from Fluka and used as received.
10.
Triethylamine (= 99.5%) was purchased from Aldrich and used as received.
11.
3,3-Dimethylbutyryl chloride (99%) was purchased from Aldrich and used as received.
12.
Hexanes (98.5%) was purchased from Aldrich and used as received.
13.
Aldrich (200-400 mesh) silica gel was used as received. The silica gel is packed in the funnel from a slurry in hexanes. The surface of the packed silica is covered with filter paper to prevent disruption.
14.
Upon concentration of the solution, a white precipitate forms.
15.
After the rinse is complete, thin layer chromatography (TLC) is used to check the flow-through for the presence of compound
1. The filter cake is rinsed with 6 × 100 mL portions of hexanes/diethyl ether (19:1) until TLC of the flow-through reveals the absence of
1.
16.
The physical properties are as follows:
R
f = 0.53 (hexanes/ethyl acetate, 19:1); IR:
2964, 2906, 2870, 1959, 1716, 1463, 1413, 1365, 1286, 1255, 1240, 1152, 1095, 1039, 874, 803 cm
−1.
1H NMR
pdf (400 MHz, CDCl
3) δ: 1.12 (s, 9 H); 1.27 (t,
J = 7.1 Hz, 3 H), 4.11-4.25 (m, 2 H), 5.59-5.63 (m, 2 H)
.
13C NMR
pdf (100 MHz, CDCl
3) δ: 14.1, 29.9, 32.5, 60.5, 89.6, 106.6, 166.1, 210.4
. HRMS [C
10H
16O
2 + H]
+: calcd. for 169.1223, found 169.1220. Anal. calcd. for C
10H
16O
2: C, 71.39; H, 9.59. Found: C, 70.79; H, 9.75.
17.
Differential scanning calorimetry of (
1) was completed (DSC, 30 °C to 300 °C, ramp 10 °C/min). Exothermic decomposition was observed with an onset temperature of 90 °C. Heating of the product beyond 30 °C and purification by distillation is therefore not recommended without further safety testing.
18.
(E)-N-Benzylidene-4-methylbenzenesulfonamide
was prepared as previously described.
2
19.
Benzene (=99.5%) was purchased from Fluka and used as received.
20.
Tributylphosphine (97%) was purchased from Aldrich and used without purification.
31P NMR spectroscopy revealed that the reagent comprised 91% tributylphosphine (d -30.65 ppm) and less than 5% tributylphosphine oxide (d 48.49 ppm). Three other resonances (d -17.35, <3%; d 34.79, <1%; d 132.33, <2%) were also observed.
21.
The mixture slowly transforms from clear to yellow after the addition of ethyl 5,5-dimethylhexa-2,3-dienoate (
1).
22.
The reaction is monitored using TLC (hexanes/ethyl acetate, 3:1), observing the disappearance of
(E)-N-benzylidene-4-methylbenzene-sulfonamide
(
R
f = 0.54) and the appearance of the product, ethyl 5-(
tert-butyl)-2-phenyl-1-tosyl-3-pyrroline-3-carboxylate (
2) (
R
f = 0.66). Visualization is performed under UV light and through staining with potassium permanganate (prepared by dissolving 20 g of potassium carbonate in 300 mL of water followed by the sequential addition of 5 mL of 5% NaOH
(aq) and 3 g of KMnO
4). Potassium permanganate was purchased from Fisher and used as received. The reaction typically reaches completion within 4-7 h. The reaction was checked for completion by TLC after 18 h prior to work-up.
23.
If a vacuum of 0.1 mmHg is not reached, the oil can be dried overnight under vacuum (20-30 mmHg), then concentrated from
diethyl ether (2 × 50 mL) and
hexanes (50 mL) to provide a viscous brown oil.
24.
The reproducibility of the crystallization is improved by generating a small amount of seed material through column chromatography.
25.
Column chromatography was performed using a 40 mm-wide glass column packed with Aldrich (200-400 mesh) silica gel (90 g). The column is packed from a slurry of silica gel in hexanes/ethyl acetate (9:1). The crude residue is loaded onto the column in the oily state obtained immediately after rotary evaporation. The flask is rinsed twice with 2 mL portions of hexanes/ethyl acetate (9:1). The column is eluted with hexanes/ethyl acetate (9:1) and fractions are collected. Each collected fraction is analyzed using TLC, and those containing the product are collected, concentrated under rotary evaporation (30 °C, 20-25 mm Hg), and dried under vacuum to afford an oil that crystallized on standing.
26.
The submitters obtained additional product from the filtrates using column chromatography
(Note 27). The checkers did not isolate additional product from the filtrate, but determined by quantitative HPLC analysis that an addition 960 mg of
2 was present in the combined mother liquors and wash.
27.
Any small amounts of solid left on the filter funnel are dissolved in ethyl acetate and combined with the filtrate prior to concentrating. The filtrate is concentrated under rotary evaporation (35 °C, 20-25 mmHg) and purified through column chromatography to yield an additional 1.0 g (7.8%) of
2 as a white solid. Column chromatography was performed using a 4.75-cm-wide glass column packed with Aldrich (200-400 mesh) silica gel (65 g). The column is packed from a slurry of silica gel in hexanes/ethyl acetate (9:1). The crude residue is loaded onto the column in the oily state obtained immediately after rotary evaporation. The flask is rinsed twice with 2 mL portions of hexanes/ethyl acetate (9:1). The column is eluted with hexanes/ethyl acetate (9:1) and fractions of ca. 22 mL are collected. Each collected fraction is analyzed using TLC and those containing the product are collected, concentrated under rotary evaporation (30 °C, 20-25 mmHg), and dried under vacuum.
28.
The physical properties are as follows: mp 93-98 °C; IR: 2957, 1714, 1335, 1232, 1164, 1102, 1089, 980 cm
−1.
1H NMR
pdf (400 MHz, CDCl
3) δ: 0.80 (s, 9 H), 1.14 (t,
J = 7.1 Hz, 3 H), 2.40 (s, 3 H), 4.12 (q,
J = 7.1 Hz, 2 H), 4.37 (d,
J = 2.3 Hz, 1 H), 5.89 (s, 1 H), 6.73 (dd,
J = 1.2, 2.5 Hz, 1 H), 7.32-7.23 (comp, 5 H), 7.43 (d,
J = 7.4 Hz, 2 H), 7.71 (d,
J = 8.2 Hz, 2 H)
.
13C NMR
pdf (100 MHz, CDCl
3) δ: 14.0, 21.5, 27.9, 35.9, 60.8, 68.4, 77.8, 127.5, 127.9, 128.0, 129.6, 134.1, 134.2, 139.7, 141.2, 143.8, 162.7
. HRMS [C
24H
29NO
4S
+ H]
+: calcd. for 428.1890, found 428.1890. Anal. calcd. for C
24H
29NO
4S: C, 67.42; H, 6.84; N, 3.28. Found: C, 67.51; H, 7.14; N, 3.20.
Handling and Disposal of Hazardous Chemicals
The procedures in this article are intended for use only by persons with prior training in experimental organic chemistry. All hazardous materials should be handled using the standard procedures for work with chemicals described in references such as "Prudent Practices in the Laboratory" (The National Academies Press, Washington, D.C., 2011 www.nap.edu). All chemical waste should be disposed of in accordance with local regulations. For general guidelines for the management of chemical waste, see Chapter 8 of Prudent Practices.
These procedures must be conducted at one's own risk. Organic Syntheses, Inc., its Editors, and its Board of Directors do not warrant or guarantee the safety of individuals using these procedures and hereby disclaim any liability for any injuries or damages claimed to have resulted from or related in any way to the procedures herein.
3. Discussion
The use of nucleophilic tertiary phosphines as catalysts in organic transformations has become a powerful tool for organic synthesis over the past two decades. The discovery that the zwitterions generated from the addition of a phosphine to an electron-deficient allene or alkyne can engage with various electrophiles to produce annulated products has generated much interest in the development of related reactions.
3 This flurry of activity is due, in part, to the complexity that can be generated from relatively simple starting materials, as well as the simplicity of the experimental procedures. For instance, workup typically requires no more that rotary evaporation and column chromatography. Most of the developed reactions have entailed the preparation of carbo- and heterocyclic products from simple starting materials, such as olefins, allenes, or acetylenes in combination with alkenes, imines, aldehydes, or dinucleophiles.
4 In the first examples of such reactions, reported by Xiyan Lu, activated allenes were reacted with electron-deficient alkenes and imines to produce cyclopentenes and pyrrolines, respectively.
5 Since Lu's original report of such [3 + 2] annulations, many enantioselective variants of the procedure have been described, as well as several applications in the realm of natural product synthesis.
6,
7 Our laboratory discovered that a-alkyl allenoates behave as 1,4-dipole-like species in novel [4 + 2] annulations with imines to form tetrahydropyridines.
8 This broadly applicable reaction has since been applied to natural product synthesis
9 and has been developed into an asymmetric process.
10 The utility of the phosphine-catalyzed [4 + 2] annulation was further enhanced upon the successful application of activated alkenes to form functionalized cyclohexenes
11 and of trifluoromethyl ketones to form dihydropyrans.
12 Of particular importance to this discussion is the synthesis of 2,5-disubstituted pyrroline-3-carboxylates through the reactions of γ-substituted allenoates and imines.
13 2,5-Disubstituted pyrroline-3-carboxylates are important scaffolds that are commonly used as intermediates en route to other important nitrogen atom-containing heterocycles.
14 Aside from their use as synthetic intermediates, some 2,5-disubstituted-3-pyrrolines themselves exhibit significant biological activity.
15 Recently, our laboratory synthesized a library of 2,5-disubstituted pyrroline-3-carboxylates through the phosphine-catalyzed [3 + 2] annulations of resin-bound allenoates and imines. Several of these compounds were identified as geranylgeranyltransferase type-I (GGTase-1) inhibitors (GGTIs) and one, P61A6, exhibits excellent antitumor effects when tested in mice.
Figure 1. Geranylgeranyltransferase type-I inhibitor P61A6.
Employing the procedure described above provides access to various 2,5-disubstituted pyrroline-3-carboxylates through the reactions of imines and 4-alkyl-2,3-butadienoates in the presence of a catalytic amount of tributylphosphine. Table 1 reveals that the reactions of various 4-alkyl- and 4-aryl-2,3-butadienoates all led to the desired 2,5-disubstituted pyrrolines in excellent yields (Table 1, entries 1-6). The process is highly diastereoselective, with preferential formation of the syn isomer ranging from 91:9 for the
γ-methyl group (Table 1, entry 1) to exclusive for
γ-isopropyl,
tert-butyl, and phenyl groups (Table 1, entries 4-6). Note that product
2c (Table 1, entry 3) was formed in a similar reaction employing the 2-butynoate, but in only 63% yield.
5b
Table 1. Synthesis of Pyrrolines 2 From γ-Substituted Allenoates
a
The scope of the reaction was further established by reacting γ-substituted allenoates with various aryl imines (Table 2). The reactions of γ-isopropyl allenoate provided the corresponding 3-pyrrolines in excellent yields (Table 2, entries 1-3). The reactions of γ-tert-butyl allenoate with various aryl imines led to quantitative yields of all products (Table 2, entries 9-16).
Table 2. Reactions of γ-Substituted Allenoates With Aryl Imines
a
Scheme 1 presents a proposed mechanism for the formation of 2,5-disubstituted-3-pyrrolines from γ-substituted allenoates and imines. Nucleophilic addition of the phosphine catalyst to the allenoate 1 results in the intermediate zwitterion 4, which then adds to the imine 5 to produce the phosphonium amide zwitterion 6. A 5-endo-trig cyclization of the amide anion to the vinyl phosphonium cation results in the formation of the ylide 7. After proton transfer to generate the β-phosphonium enolate intermediate 8, liberation of the catalyst, through β-elimination of the phosphine, yields the final 2,5-disubstituted-pyrroline-3-carboxylate (2).
Scheme 1. Proposed Mechanism for the Phosphine-Catalyzed [3 + 2] Annulations of γ-Substituted Allenoates and Imines
In summary, various 2,5-disubstituted 3-pyrrolines are readily available from the reactions of γ-substituted allenoates with aryl imines in the presence of catalytic amounts of a trialkylphosphine. These phosphine-catalyzed reactions are highly efficient, diastereoselective, operationally simple, and executed under mild conditions.
Appendix
Chemical Abstracts Nomenclature (Collective Index Number);
(Registry Number)
Ethyl 5,5-dimethylhexa-2,3-dienoate: 2,3-Hexadienoic acid, 5,5-dimethyl-, ethyl ester; (35802-59-6)
Ethyl (triphenylphosphoranylidene)acetate: Acetic acid, 2-(triphenylphosphoranylidene)-, ethyl ester; (1099-45-2)
3,3-Dimethylbutyryl chloride: Butanoyl chloride, 3,3-dimethyl-; (7065-046-05)
Triethylamine: Ethanamine, N,N-diethyl-; (121-44-8)
Ethyl 5-(tert-butyl)-2-phenyl-1-tosyl-3-pyrroline-3-carboxylate: 1H-Pyrrole-3-carboxylic acid, 5-(1,1-dimethylethyl)-2,5-dihydro-1-[(4-methylphenyl)sulfonyl]-2-phenyl-, ethyl ester, (2R,5R)-rel-; (861668-04-4)
(E)-N-Benzylidene-4-methylbenzenesulfonamide: Benzenesulfonamide, 4-methyl-N-(phenylmethylene)-, [N(E)]-; (51608-60-7)
Tributylphosphine; (9
98-40-3)
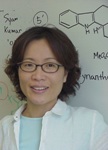 |
Ohyun Kwon was born in South Korea in 1968. She received her B.S. and M.S. in chemistry (with Eun Lee) from Seoul National University in 1991 and 1993, respectively. She came to the US in 1993 and obtained her PhD (with Samuel J. Danishefsky) from Columbia University in 1998. After a postdoctoral stint in the laboratory of Stuart L. Schreiber at Harvard University, she began her independent career in 2001 as an Assistant Professor at the University of California, Los Angeles (UCLA). In 2008, she was promoted to Associate Professor with tenure at UCLA. Her research involves the development of phosphine-catalyzed reactions and their application to natural product synthesis and chemical biology. |
 |
Ian P. Andrews was born in Monterey, California in 1983. He received his B.S. degree from the University of California, San Diego in 2005. He was a summer intern at Pfizer inc. working in medicinal chemistry on the discovery of new carbonic anhydrase inhibitors. He worked at Trinlink Biotechnologies in San Diego before pursuing a Ph.D. at the University of California, Los Angeles. He is currently conducting research in the Laboratory of Dr. Ohyun Kwon at UCLA. |
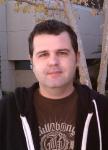 |
John T. Colyer earned his B.S. in chemistry from Indiana University-Purdue University Indianapolis in 2000 working under the guidance of Professor William H. Moser. He completed his M.S. in 2004 at the University of Arizona under the guidance of Professor Michael P. Doyle, where he studied dirhodium (II) carboxamidate catalysis. In 2004 he joined the Chemical Process Research & Development department at Amgen in Thousand Oaks, CA. |
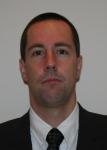 |
Oliver R. Thiel studied chemistry at the Technical University Munich, Germany and completed a Diploma thesis on rhodium-catalyzed hydroaminations under supervision of Professor Matthias Beller. He then pursued a Ph.D. (1998-2001) at the Max-Planck-Institut für Kohlenforschung Mülheim, Germany under guidance of Professor Alois Fürstner, exploring RCM-reactions in natural product synthesis. After a postdoctoral appointment (2001-2003) at Stanford University with Professor Barry M. Trost, Oliver joined the Chemical Process Research & Development department at Amgen in Thousand Oaks, CA where he has been involved in the development of synthetic processes of numerous clinical candidates. |
Copyright © 1921-, Organic Syntheses, Inc. All Rights Reserved