Org. Synth. 2011, 88, 247-259
DOI: 10.15227/orgsyn.088.0247
LITHIATED PRIMARY ALKYL CARBAMATES FOR THE HOMOLOGATION OF BORONIC ESTERS
Submitted by Matthew P. Webster, Benjamin M. Partridge, and Varinder K. Aggarwal.
1.
Checked by Tomohiko Inui and Tohru Fukuyama.
1. Procedure
A. N,N-Diisopropyl-carbamic acid 3-phenyl-propyl ester (1). A 1 L flask containing a 40 mm magnetic stirring bar is charged with 3-phenylpropan-1-ol (Note 1, 51.0 mL, 51.1 g, 375 mmol, 1.00 equiv), N,N-diisopropylcarbamoyl chloride (Note 1, 62.7 g, 383 mmol, 1.02 equiv), triethylamine (Note 1, 53.4 mL, 38.8 g, 383 mmol, 1.02 equiv) and dichloromethane (Note 1, 500 mL). A reflux condenser is fitted and connected to an argon line by way of a gas inlet adaptor. The reaction mixture is stirred and heated at reflux for 24 h. After cooling to room temperature, the solvent is removed in vacuo (200 mmHg, 30 °C) using a rotary evaporator and tert-butyl methyl ether (Note 2, 500 mL) is added. The mixture is transferred to a separatory funnel and washed with water (2× 400 mL), and the combined aqueous phases are extracted with tert-butyl methyl ether (2× 300 mL). The combined organic fractions are washed with brine (100 mL) and dried over MgSO4 (15 g) for 2 min. After filtration of the solids, the filtrate is concentrated in vacuo (100 mmHg, 30 °C) to give a pale yellow oil. The crude material is transferred to a 250 mL flask equipped with an oval-shaped, Teflon coated, 40 mm magnetic stirring bar. This is fitted with a short path distillation kit incorporating a thermometer. The crude carbamate is distilled (~0.6 mmHg, 122-123 °C) to give the analytically pure N,N-diisopropyl-carbamic acid 3-phenyl-propyl ester (83.2-86.3 g, 84-87%) as a colorless oil (Note 3).
B. Isobutylboronic acid pinacol ester (2). An oven-dried 1 L flask containing a 40 mm magnetic stirrer bar is charged with isobutylboronic acid (4, 25.0 g, 245 mmol 1.0 equiv), pinacol (Note 4, 29.0 g, 245 mmol, 1.0 equiv), magnesium sulfate (Note 4, 44.3 g, 368 mmol, 1.5 equiv) and diethyl ether (Note 4, 300 mL). The suspension is stirred under argon at room temperature for 24 h. After filtration of the solids, the filtrate is concentrated in vacuo (200 mmHg, 30 °C). The crude material is dissolved in pentane (Note 5, 700 mL) and washed with H2O (3× 150 mL), and the organic layer is dried over MgSO4. After filtration of the solids, the filtrate is concentrated in vacuo (200 mmHg, 30 °C) to give a pale yellow oil. The crude material is transferred to a 250 mL flask equipped with a 40 mm magnetic stirring bar. This is fitted with a short path distillation kit incorporating a thermometer. The crude boronic ester is distilled (14 mmHg, 71 °C) to give the analytically pure isobutylboronic acid pinacol ester (35.3-37.4 g, 78-83%) as a colorless oil (Note 7).
C. (R)-5-Methyl-1-phenylhexan-3-ol (3). An oven-dried 1 L three-necked flask equipped with a 40 mm magnetic stirring bar, an oven dried 250 mL pressure equalising dropping funnel, a thermocouple (Note 8) and a gas inlet adaptor is attached to a vacuum manifold. The reaction flask is evacuated and refilled with argon (× 2). Carbamate 1 (13.2 mL, 13.2 g, 50.0 mmol, 1.0 equiv), (-)-sparteine (Note 9, 14.9 mL, 15.2 g, 65.0 mmol, 1.3 equiv, addition by syringe) and anhydrous diethyl ether (Note 10, 250 mL, addition via cannula) are charged to the reaction vessel and the resulting solution is cooled to -78 °C (dry ice/acetone bath). A solution of sec-butyllithium (11, 61.3 mL, 1.06 M in cyclohexane/hexane (95/5), 65.0 mmol, 1.3 equiv) is added to the reaction mixture via dropping funnel over ~30 min whilst maintaining an internal temperature below -70 °C. The reaction mixture is then stirred below -70 °C for 5 h (Note 12). Neat boronic ester2 (13.9 mL, 12.0 g, 65.0 mmol, 1.3 equiv) is added drop-wise using a syringe over 10 min whilst maintaining an internal temperature below -70 °C. The reaction mixture is then stirred below -70 °C for 30 min before being removed from the cooling bath and allowed to warm to room temperature (Note 13). The center neck of the flask is fitted with an oven-dried reflux condenser attached to an argon line by a gas inlet adaptor. The additional necks of the flask are secured with glass stoppers, and the reaction mixture is heated at reflux for >12 h (Note 14). The reaction mixture is allowed to cool to room temperature and the condenser exchanged with a 250 mL dropping funnel. After being cooled in an ice/water bath, a mixture of H2O2 (Note 15, 50 mL, 30 % w/v) and NaOH (100 mL, 2 M) is added via dropping funnel over 5 min. The vessel is removed from the cooling bath, and the resulting mixture is stirred at room temperature for 2 h (Note 16). Sodium hydroxide (200 mL, 2 M) is added, and the mixture is partitioned in a separatory funnel. The aqueous phase is extracted with diethyl ether (2× 300 mL), and the combined organic fractions are washed with KHSO4 (200 mL, 1 M), H2O (2× 400 mL), brine (100 mL) and dried over MgSO4 (10 g) for 2 min. After filtration of the solids, the filtrate is concentrated in vacuo (150 mmHg, 30 °C) to give a pale yellow oil. The crude material is transferred to a 50 mL flask equipped with a 25 mm magnetic stirring bar. This is fitted with a short path distillation kit incorporating a thermometer. The crude alcohol is distilled (1.2-1.3 mmHg, 101-103 °C) to give the analytically pure alcohol3 (7.86-8.34 g, 82-87%, e.r. = 99:1 (Note 17)) as a colorless oil (Note 18).
2. Notes
1.
3-Phenylpropan-1-ol and
N,N-diisopropylcarbamoyl chloride were obtained from Sigma-Aldrich, whereas
triethylamine (>99.0%) and
dichloromethane (>99.5%) were obtained from Kanto Chemical Co., Inc.. The Submitters obtained triethylamine and dichloromethane (Laboratory Reagent Grade) from Fischer Scientific. All reagents were used as purchased.
2.
tert-Butyl methyl ether (Reagent Grade) was obtained from Sigma-Aldrich.
3.
In accordance with the literature,
2 the product exhibits the following physiochemical properties:
1H NMR
pdf(CDCl
3, 400 MHz) δ: 1.22 (d,
J = 6.9 Hz, 4× CH
3, 12 H), 1.98 (tt,
J = 7.8, 6.4 Hz, ArCH
2C
H2, 2 H), 2.72 (t,
J = 7.8 Hz, ArCH
2, 2 H), 3.40-4.35 (br. m, NCH, 2 H), 4.12 (t,
J = 6.4 Hz, OCH
2, 2 H), 7.16-7.25 (m, ArH, 3 H), 7.25-7.33 (m, ArH, 2 H);
13C NMR
pdf(CDCl
3, 100 MHz) δ: 21.0 (br), 30.9, 32.5, 45.8 (br), 64.0, 125.9, 128.3, 128.4, 141.5, 155.8; HRMS (ESI) calcd. for C
16H
25NNaO
2: 286.1783. Found: 286.1783; IR
νmax (neat) 2969, 1692, 1436, 1368, 1309, 1290, 1219, 1157, 1134, 1066 cm
-1; Anal. Calcd. for C
16H
25NO
2: C, 72.96; H, 9.57; N, 5.32. Found: C, 72.93; H, 9.56; N, 5.37; Submitters reported: MS (EI): 263 (5, M
+), 118 (78), 91 (100).
4.
Isobutylboronic acid was obtained from Frontier Scientific, pinacol was obtained from
Alfa-Aesar, MgSO4 (>95.0%) and
diethyl ether (>99.0%) were obtained from Wako Pure Chemical Industries, Ltd. The Submitters obtained MgSO
4 from Fischer Scientific and diethyl ether (Laboratory Reagent Grade) from VWR. All reagents were used as purchased.
5.
Pentane (>97.0%) was obtained from Wako Pure Chemical Industries, Ltd. and used as purchased. The Submitters obtained pentane (Laboratory Reagent Grade) from Fischer Scientific.
6.
The Submitters note that the boronic ester sublimes at lower temperatures so the material should be heated rapidly to prevent loss of material.
7.
In accordance with the literature,
3 the product exhibits the following physiochemical properties :
1H NMR
pdf(CDCl
3, 400 MHz), δ: 0.73 (d,
J = 7.3 Hz, CH
2, 2 H), 0.93 (d,
J = 6.4 Hz, 2× CHC
H3, 6 H), 1.25 (s, 4× CCH
3, 12 H), 1.86 (t·septet,
J = 7.3, 6.4 Hz, CH, 1 H);
13C NMR
pdf(CDCl
3, 100 MHz) δ: 24.81, 24.81 (4C), 24.85, 25.2, 82.8; HRMS(DART) calcd. for C
10H
22BO
2: 185.1713. Found: 185.1712; IR
νmax (neat) 2979, 2954, 1371, 1312, 1209, 1146, 972, 848 cm
-1; Anal. calcd. for C
10H
21BO
2: C, 65.25; H, 11.50. Found: C, 64.86; H, 11.36; Submitters reported:
11B NMR (CH
2Cl
2, 128 MHz) δ: 33.9; MS (CI): 185 (17, MH
+), 141 (55), 85 (100).
8.
Internal temperature was monitored using a CUSTOM thermocouple (CT-470) fitted with K-type flexible wire probe (passed through a septum). The Submitters used a Hanna thermocouple (HI 93531).
9.
(-)-Sparteine was obtained from the commercially available
sulfate salt (>98.0%, Wako Pure Chemical Industries, Ltd.) according to the literature procedure.
4 The submitters obtained (-)-sparteine from Sigma-Aldrich, which was distilled from CaH
2 under high vacuum prior to use (~0.1 mmHg, 98-100 °C, colorless oil). The submitters note that distilled (-)-sparteine can be stored and used for more than 6 months if stored correctly, i.e. in appropriate Schlenk glassware under argon at -20 °C.
10.
Anhydrous diethyl ether was obtained using a Grubbs-type system.
5
11.
Solutions of
sec-butyllithium were purchased from
Kanto Chemical Co., Inc. The submitters obtained solutions of
sec-butyllithium from Acros Organics. The submitters note that the yields of the reactions are critically dependant on the quality of the
sec-butyllithium. The best results are obtained with fresh solutions (up to 2 months shelf life) that have been stored in a refrigerator and which are colorless to pale yellow in color with minimal precipitated salts. See also Beak,
et al.
4 The submitters note that 1.3 equiv of
sec-butyllithium is used with respect to carbamate in the homologation reactions as occasionally lower yields were obtained with less reagent.
12.
During the addition of
sec-butyllithium, a yellow coloration is immediately apparent and increases in intensity over the lithiation period.
13.
The yellow color observed during lithiation reduces upon warming to room temperature.
14.
After heating at reflux overnight a pale yellow solution is produced.
15.
Hydrogen peroxide was obtained from Kanto Chemical Co., Inc. The submitters obtained hydrogen peroxide from Fischer Scientific.
16.
Oxidation can be monitored by TLC after partitioning of an aliquot in H
2O/diethyl ether. Eluent: ethyl acetate/hexane, 1:9.
Rf boronic ester = 0.7, alcohol = 0.3 (Submitters: Eluent: ethyl acetate/petroleum ether 40-60 °C, 1:9.
Rf boronic ester = 0.7, alcohol = 0.2). TLC plate visualized using
phosphomolybdic acid [5% (NH4)2Mo7O24•4H2O in 95% EtOH (w/v)] followed by heating.
17.
Chiral HPLC conditions for separation of alcohol (R)- 3. Checkers: Column: Daicel Chiralpak AD-H (250 × 4.6 mm) with Chiralpak AD-H Guard Cartridge (10 × 4 mm), eluent: 99.0:1.0 hexane:propan-2-ol (both HPLC grade,
hexane was purchased from Wako Pure Chemical Industries, Ltd.,
propan-2-ol was purchased from Kanto Chemical Co., Inc.), flow rate: 0.7 mL/min, t
R: minor (
S) 22.4 min, major (
R) 24.2 min. (Submitters: Column: Daicel Chiralpak IA (250 × 4.6 mm) with Chiralpak IA Guard Cartridge (10 × 4 mm), eluent: 97.5:2.5 hexane:propan-2-ol (both HPLC grade, purchased from Fisher Scientific), flow rate: 0.7 mL/min, t
R: minor (
S) 16.6 min, major (
R) 17.8 min.)
18.
In accordance with the literature,
6 the product exhibits the following physiochemical properties.
1H NMR
pdf(CDCl
3, 400 MHz) δ: 0.90 (d,
J = 6.0 Hz, CH
3, 3 H), 0.92 (d,
J = 6.4 Hz, CH
3, 3 H), 1.28 (m, CH(OH)CH
HCH(CH
3)
2, 1 H), 1.35 (br. s, OH, 1 H), 1.42 (m, CH(OH)C
HHCH(CH
3)
2, 1 H), 1.65-1.87 (m, C
H + PhCH
2C
HH, 3 H), 2.67 (ddd,
J 13.7, 9.6, 6.4 Hz, PhCH
H, 1 H), 2.80 (ddd,
J 13.7, 10.1, 6.0 Hz, PhC
HH, 1 H), 3.65-3.77 (m, C
HOH, 1 H), 7.14-7.36 (m, ArH, 5 H);
13C NMR
pdf(CDCl
3, 100 MHz) δ: 22.1, 23.4, 24.6, 32.0, 39.7, 46.8, 69.5, 125.8, 128.4 (4C), 142.2; HRMS(ESI) calcd. for C
13H
20NaO: 215.1412. Found: 215.1416; IR
νmax (neat) 3349, 2954, 2927, 1496, 1467, 1455, 1367, 1055 cm
-1; Anal. Calcd. for C
13H
20O: C, 81.20; H, 10.48. Found: C, 80.91; H, 10.58; [a]
D21 +4.7 (
c 1.10, CHCl
3), lit. +1.2 (
c 1.00, CHCl
3); Submitters reported: MS (CI): 175 (27, MH
+ - OH), 91 (77), 85 (100).
Safety and Waste Disposal Information All hazardous materials should be handled and disposed of in accordance with "Prudent Practices in the Laboratory"; National Academy Press; Washington, DC, 1995.
3. Discussion
The 1,2-metallate rearrangement of ate-complexes derived from boranes and boronic esters has been used by several groups to produce organoboranes and boronic esters with high enantiomeric purity.
7 The seminal work of Matteson demonstrated the zinc chloride-mediated homologation of boronic esters using dichloromethyllithium. In these examples, asymmetry is introduced by the use of an enantiopure chiral diol (substrate control, Scheme 1) to provide, after 1,2-metallate rearrangement,
α-chloroboronic esters with very high diastereomeric purity.
8 Reaction with an alkyl metal provides the corresponding secondary boronic esters with high diastereo- and enantioenrichment.
Scheme 1. Matteson-type substrate controlled homologation.
Chiral carbenoids have also been used for the homologation of achiral boranes/boronic esters to give chiral organoboranes and boronic esters (reagent control, Scheme 2). Such reagents include enantioenriched sulfur ylides,
9 Hoffmann-type
10α-chloroalkylmetal derivatives,
6,11 lithiated epoxides,
12 lithiated aziridines
13 and
N-linked carbamates.
14 All have been reported to be highly effective providing the corresponding products in high yields and with excellent levels of stereocontrol.
Scheme 2. Reagent controlled homologation.
The direct reaction of lithiated Hoppe-type carbamates
15 with boranes and boronic esters provides, after 1,2-metallate rearrangement and oxidation, secondary alcohols of high enantiopurity (Table 1).
16 This reaction shows excellent substrate scope and was evaluated for a range of carbamates and boron reagents. Moreover, either enantiomer of the lithiated carbamate is easily prepared by simple choice of (-)-sparteine or O'Brien's (+)-sparteine surrogate.
17
In this procedure we demonstrate the robust nature of this transformation for the homologation of alkyl boronic esters. As previously reported
18 we note that MgBr
2 is not required to activate the rearrangement for the homologation of alkyl substituted boronic esters. The homologation of phenyl pinacol boronic ester with carbamate
1 has also been achieved on a 50 mmol scale within our laboratory, though not reported herein as a checked procedure. Unlike the case with alkyl boronic esters, in this case it was found that MgBr
2 was required to promote the 1,2-metallate rearrangement (Scheme 3).
Table 1. Homologation using Hoppe's lithiated carbamates.
|
Entry
|
Carbamate
|
R2
|
(R3)2
|
L.A.
|
Yield (15) [%]
|
e.r.
|
|
1
|
12aEt
|
Et
|
Et
|
-
|
91
|
98:2
|
2
|
|
nHex
|
9-BBN
|
-
|
90
|
98:2
|
3
|
|
iPr
|
9-BBN
|
-
|
81
|
98:2
|
4
|
|
Ph
|
9-BBN
|
-
|
85
|
88:12
|
5
|
|
Ph
|
9-BBN
|
MgBr2
|
94
|
97:3
|
6
|
|
Et
|
pinacol
|
MgBr2
|
90
|
98:2
|
7
|
12b
|
Et
|
Et
|
-
|
90
|
97:3
|
8
|
|
Ph
|
9-BBN
|
MgBr2
|
71
|
95:5
|
9
|
|
Et
|
pinacol
|
MgBr2
|
75
|
97:3
|
10
|
|
Ph
|
pinacol
|
MgBr2
|
73
|
98:2
|
11
|
12c
|
Et
|
Et
|
-
|
67
|
95:5
|
12
|
|
Ph
|
9-BBN
|
MgBr2
|
65
|
97:3
|
13
|
|
Ph
|
pinacol
|
MgBr2
|
64
|
98:2
|
14
|
12d
|
Ph
|
9-BBN
|
MgBr2
|
68
|
96:4
|
15
|
|
Ph
|
pinacol
|
MgBr2
|
70
|
98:2
|
16
|
12e
|
Ph
|
pinacol
|
MgBr2
|
70
|
97:3
|
|
Scheme 3. Homologation of phenyl pinacol boronic ester with carbamate 1.
It has also been reported that reagent-controlled, multiple homologations of a boronic ester are possible
16a,18 and that these can be carried out in a one-pot fashion without detriment to selectivity.
18 This process has been likened to a molecular assembly line in which successive groups are added to a growing chain with control of relative and absolute stereochemistry.
The stereodivergent use of lithiated chiral secondary benzylic carbamates
19 for the homologation of organoboranes/boronic esters has also been reported.
20 Remarkably, choice of organoborane or boronic ester allows, after oxidation, access to either enantiomer of the tertiary alcohol product from the same enantiomer of carbamate. Excellent yield and chirality transfer was observed in all cases. The benzylic boronic ester products have been isolated, shown to undergo effective protodeboronation
21 and conversion to products bearing all carbon quaternary centers.
22
The intermediate boron compounds can also be converted to their amine
23 or trifluoroborate counterparts.
23c Both secondary and tertiary trifluoroborate compounds are easily prepared and have been reported to undergo Rh catalysed
24 and Lewis-acid catalysed
24b 1,2-addition to aldehydes with almost complete retention of stereochemistry.
Appendix
Chemical Abstracts Nomenclature (Collective Index Number);
(Registry Number)
N,N-Diisopropyl-carbamic acid 3-phenyl-propyl ester; (218601-55-9)
Isobutylboronic acid pinacol ester; (67562-20-3)
(R)-5-Methyl-1-phenylhexan-3-ol; (481048-36-6)
3-Phenylpropan-1-ol; (122-97-4)
N,N-Diisopropylcarbamoyl chloride; (19009-39-3)
Triethylamine; (121-44-8)
Isobutylboronic acid: Boronic acid, B-(2-methylpropyl)-; (84110-40-7)
Pinacol; (79-09-5)
sec-Butyllithium; (598-30-1)
(-)-Sparteine; (90-39-1)
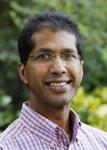 |
Varinder K. Aggarwal was born in Kalianpur in North India in 1961 and emigrated to the United Kingdom in 1963. He received his B.A. (1983) and Ph.D. (1986) from Cambridge University, the latter under the guidance of Dr Stuart Warren, and carried out postdoctoral work with Professor Gilbert Stork at Columbia University, NY (1986-1988). He is currently at the University of Bristol where he has held the Chair of Synthetic Chemistry since 2000. His research interests include the development of novel asymmetric synthetic methodology, catalysis and total synthesis. |
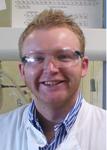 |
Matthew P. Webster was born in Bridlington, UK, in 1981. He received a masters degree in chemistry at the University of Hull, UK, in 2005 and subsequently joined the group of Prof. Aggarwal at the University of Bristol. He obtained his Ph.D. in 2010 on the multiple homologations of boronic esters and applications in synthesis. Since January 2010 he has been a postdoctoral scholar in the group of Prof. Alois Fürstner at the Max-Planck-Institut, Mülheim, Germany. |
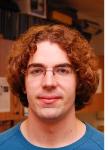 |
Benjamin M. Partridge was born in Birmingham, UK, in 1985. He graduated in 2007 with a masters degree in chemistry from the University of Bristol. Currently, he is pursuing a Ph.D. degree under the direction of Prof. Aggarwal focussed on the asymmetric total synthesis of medicinally relevant compounds. |
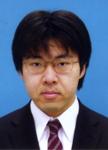 |
Tomohiko Inui was born in Osaka, Japan in 1983. He received his B.S. in 2006 from Kyoto University under the direction of Professor Tamejiro Hiyama. He then moved to the laboratories of Professor Tohru Fukuyama, the University of Tokyo. He received his M.S. in 2008 and he is pursuing a Ph.D. degree. His current research interest is total synthesis of natural products. |
Copyright © 1921-, Organic Syntheses, Inc. All Rights Reserved