Org. Synth. 2011, 88, 260-290
DOI: 10.15227/orgsyn.088.0260
Discussion Addendum for: PALLADIUM-CATALYZED REDUCTION OF VINYL TRIFLUOROMETHANESULFONATES TO ALKENES: CHOLESTA-3,5-DIENE
Submitted by Sandro Cacchi, Enrico Morera, and Giorgio Ortar.
1.
Discussion
Ever since our first reports describing the palladium-catalyzed reduction of vinyl
2 and aryl
3 triflates to alkenes and arenes, this methodology has found a large number of applications ranging from the preparation of fine chemicals to the synthesis of biologically active substances.
Some modifications of the original protocol have also been developed.
The aim of the present addendum is to provide a brief updated overview of recent developments in this type of chemistry.
Reactions have been categorized into three main sections: modifications of the original conditions, reduction of vinyl triflates, and reduction of aryl triflates.
The latter two sections are further subclassified by the class of compounds used as substrates.
Modification of the Original Conditions
In general, there have been minor modifications of the original reaction conditions.
Though other solvents such as toluene,
4 THF,
5 dioxane,
6 DMSO,
7 MeOH,
8 EtOH/THF,
9 MeOH/THF,
10 EtOH,
11 AcOEt,
12 benzene,
13 MeCN,
14 and EtOH/AcOEt
15 have been used in some cases, DMF has been the solvent of choice.
In general, apart from the occasional utilization of K
2CO
3,
14 nitrogen bases have been employed and reductions have been usually carried out in the presence of Et
3N or Bu
3N.
The utilization of 2,6-lutidine
5d and EtN(
i-Pr)
2,
7,9,11,16 has also been described. As to the catalyst system, in addition to Pd(OAc)
2/PPh
3, Pd(OAc)
2/dppf [1,1'-bis(diphenylphosphino)ferrocene], and preformed Pd(OAc)
2(PPh
3)
2 originally used as precatalysts in this chemistry, reduction of organic triflates have been successfully performed in the presence of Pd(acac)
2/Bu
3P,
5 PdCl
2(PPh
3)
2,
6c,17 Pd(PPh
3)
4,
5b-e,6a,c,g,h,14,18 Pd(PPh
3)
4/dppf,
13 Pd
2(dba)
3/P(o-tol)
3,
6f Pd(OAc)
2/P(OMe)
3,
19 Pd/C,
8,9,10,11,12a,15,20 PdCl
2(dppf),
15b,21 Pd(OAc)
2/dppp [1,3-bis(diphenylphosphino)propane],
22 Pd(OAc)
2/dppb [1,3-bis(diphenylphosphino)butane],
23 Pd(OAc)
2/dppp/dppb,
7b Pd(OH)
2/C,
12 and PdCl
2(PPh
3)
2/dppp.
24 The latter combination was found to be particularly useful with electron-rich or highly hindered aryl triflates (Scheme 1).
Formic acid (in the presence of nitrogen bases) has been the reducing agent of choice in the vast majority of applications.
However, the utilization of other reducing agents has been described of late.
Aryl triflates have been reduced to arenes using HCO
2NH
4,
8,19 Et
3SiH,
5c,13,17g,18,23 H
2,
8a,9,10,11,12,15 (
n-Bu)
3SnH,
5b,d,6c Me
2NH_BH
3,
14 in the presence of Pd/C, Mg, MeOH, NH
4OAc,
8b,25 and of Pd(OAc)
2, BINAP, K
2CO
3 in DMF.
14b In some cases, pinacolborane was found to act as an hydride source in the reaction with aryl triflates.
In one of these examples, the reduction product formed readily in the presence of PdCl
2(dppf) and dioxane at room temperature.
26 With DCO
2D
27 (Scheme 2) or
n-BuSnD
5e the reduction of vinyl and aryl triflates is a powerful tool for substituting a C-D bond for a C-O bond.
An interesting variation is the development of polymer-supported triflating agent equivalents which allow for the reduction process to be performed under solid-phase conditions.
Indeed, as a result of the general acceptance of combinatorial techniques as a useful tool in drug discovery programs, there is a demand for polymer-supported reagents.
Particularly, because of the importance of making molecules lacking any extraneous functionality, traceless linkers represent an exciting aspect of solid-phase synthesis.
In this context, a couple of proposals have recently been reported which are based on the utilization of perfluoroalkyl
28 and perfluoroaryl
29 sulfonyl linkers.
An example of this chemistry is shown in Scheme 3.
28
Palladium-Catalyzed Reduction of Vinyl Triflates to Alkenes
Alkaloids
The synthesis of alkaloid derivatives has taken particular advantage of the selective palladium-catalyzed reduction of vinyl triflates.
The methodology was recently employed in the asymmetric synthesis of the 3-methyl-1-azatricyclo[5.2.1.0]decane substructure,
17f embedded within the diterpene-derived carbon scaffold of the hetisine alkaloids.
Some steps of this synthesis involve conjugate reduction of
1 and triflation of the transient enolate with PhNTf
2 to yield the corresponding
vinyl triflate in 70% yield, which was reduced to the
alkene 2 in 84% yield (Scheme 4).
Other applications in this area involve the preparation of a 'minilibrary' of analogues of manzamine A,
30 an important lead structure for the development of novel antimalarial chemotherapies, the synthesis of 2-azabicyclo[2.2.2]oct-5-enes bearing an endo alkenyl substituent,
31 a study devoted to the investigation of isoquinuclidines as precursors of certain
Gelsemium alkaloids, a divergent synthesis of tropane (8-azabicyclo[3.2.1]octane)-type alkaloid (+)- and (-)-ferruginine,
32 a concise synthesis of (-)-anatoxin-a,
33 the synthesis of the 2β,3α- and 3β,3β-isomers of 3-(
p-substituted phenyl)tropane-2-carboxylic acid methyl esters,
34 the enantioselective 20 step total synthesis of the tetracyclic spermidine alkaloid (-)-hispidospermidin,
35 a general synthetic pathway to
Strychnos indole alkaloids (-)-dehydrotubifoline and (-)-tubifoline,
16a the reduction of pyridone derivatives,
36 the synthesis of bioavailable 4,4-disubstituted piperidines,
14a the first total synthesis of the tetracyclic lactone (-)-secu'amamine A, a member of the
Securinega alkaloid family showing a wide range of biological activities,
37 the synthesis of 1,2-dihydrochromeno[3,4-
f]quinoline derivatives, selective progesterone receptor modulators (SPRM),
38 the synthesis of Calothrixins B, an antiproliferative agent from the strains of
Calothrix cyanobacterium with an indolo[3,2-
j]phenanthridine core structure,
6h a study on rearrangement of spirocyclic oxindoles, the core skeleton of several naturally occurring alkaloids isolated from
Gelsemium sempervirens,
39 the asymmetric synthesis of all the known phlegmarine alkaloids, decahydroquinolin-piperidines of significant biological activities,
40 and several syntheses of
Lycopodium alkaloids,
17c,41 a class of alkaloids containing various structural features which provides challenging targets for total synthesis.
As to the latter research area, an example is given in Scheme 5,
41a showing the reduction of the triflate
4 to the corresponding alkene.
The authors report that the conversion of the enone
3 into the corresponding triflate proved to be more challenging than anticipated.
Simple addition of the triflating reagent,
N-(5-chloro-2-pyridyl)triflimide, to the enolate formed in situ via copper-mediated 1,4-addition to
3 of the Grignard of (chloromethy1)dimethylphenylsilane failed to yield vinyl triflate
4.
Addition of DMPU followed by heating at reflux was necessary to trap the enolate.
Sphingosine
Reduction of acyclic vinyl triflates was used in key steps of the synthesis of D-
erythro-sphingosine - an unsaturated 18-carbon amino alcohol which is a primary component of glycosphingolipids and ceramids - from a chiral β-lactam obtained from D-(-)-tartaric acid
42 and from commercially available and cheap D-
ribo-phytosphingosine (Scheme 6).
43
Terpenoids and Steroids
Triflates derived from α-hydroxy-α,β-enones were selectively converted into the corresponding α,β-enones in a study devoted to the preparation of analogues of brusatol, an antitumor quassinoid, which were examined for their cytotoxic activity.
44 The reaction was used in the synthesis of (-)-homogynolide A, a bakkanolide sesquiterpene isolated from
Homogyne alipina active as insect antifeedant,
45 in the stereoselective synthesis of metasequoic acids B
, a new antifungal diterpene recently isolated from
Metasequoia glyptostroboides which has a labdane skeleton with a cyclopropane ring fused at C(3) and C(4).
Compounds with a cyclopropane moiety of this kind are rare among the diterpenoids.
46
In a study on the synthesis of C ring modified analogues of 1α,25-dihydroxyvitamin D
3,
compound 6 was prepared from 5 in 74% yield (Scheme 6).
47 Attempts to reduce
5 with Bu
3SnH or Et
3SiH in the presence of Pd(PPh
3)
4 and LiCl in THF yielded complex reaction mixtures.
48
Reduction of the vinyl triflate
7 (Scheme 8) was successfully performed under typical conditions in the 31 step asymmetric total synthesis of (-)
-merrilactone A (1.1% overall yield),
49 a sesquiterpenoid isolated from
Illicium merrillianum which was shown to possess neuroprotective and neuritogenic activity in cultures of fetal rat cortical neurons.
In the synthesis of a novel sesquiterpene isolated from the pheromone gland of a stink bug,
Tynacantha marginata Dallas, the tricyclic ketone
8 was converted to the alkene
10 via the vinyl triflate
9 (Scheme 9).
50
Enol triflate
11 has been employed as a key intermediate to access to some taiwaniaquinoids, a family of unusual tricyclic diterpenes isolated from East Asian conifers with interesting biological activities.
Palladium-catalyzed reduction of
11 gave indene
12 in excellent yield.
Because of steric hindrance, the comparatively small ligand trimethyl phosphite was required to effectively carry out his reaction (Scheme 10).
19a
Some interesting applications to steroid chemistry have also been reported.
A series of unsaturated steroids bearing a carboxy substituent at the C-3 position were prepared and assayed in vitro as inhibitors of human and rat prostatic steroid 5α-reductase.
Some of the unsaturated 3-carboxysteroids were prepared by triflation of 11-ketone derivatives followed by palladium-catalyzed reduction.
51 The procedure was used to prepare 6,7-didehydroestrogens.
52
In the synthesis of zymosterol, fecosterol, and related biosynthetic sterol intermediates,
53 multigram quantities of key intermediates containing 8,14-dienic systems were efficiently prepared from the corresponding enones though palladium-catalyzed reduction of vinyl triflates under standard conditions (Scheme 11).
53c
A selective reduction of the 17-vinyl triflate in the presence of the 3-aryl triflate was achieved using Pd(dppf)Cl
2-dichloromethane adduct as the catalyst in the synthesis of four metabolites of the antitumor drug ENMD-1198, a promising analogue of 2-methoxyestradiol for the treatment of various cancers (Scheme 12).
21e
Further applications in the area of terpenoids and steroids include a stereoselective synthesis of the CD-ring structure of cortistatin A,
54 a straightforward and scaleable synthesis of the sterically congested pheromone of the longtailed mealybug
Pseudococcus longispinus,
55 a concise diastereoselective and enantiopure route from limonene glycol to the (1
S,4
R)- and (1
S,4
S)-isomers of 4-isopropyl-1-methyl-2-cyclohexen-1-ol (aggregation pheromones of the ambrosia beetle
Platypus quercivorus),
16d and a highly regio- and stereoselective synthesis of C1 hydroxylated 19-norvitamin D analogs from the corresponding 25-hydroxyvitamin D precursors.
56
Carbocycles
A variety of olefin-containing carbocycles have been prepared from the corresponding ketonic derivatives via palladium-catalyzed reduction of vinyl triflates in the presence of formate anions.
This chemistry was applied to bicyclo[2.2.2]octene-2,5-diones,
17a,e to cyclic 1,2-diketones - used as building blocks for the asymmetric synthesis of cycloalkenones
57 - to the enantioselective total synthesis of (-)-subergorgic acid,
58 to the preparation of intermediates in the synthesis of carba-spirocyclic compounds,
59 to a concise asymmetric synthesis of hamigeran B,
60 and in a key step (Scheme 13) of a stereocontrolled functionalization of the diene system of compactin.
61
In a synthetic study devoted to the assembly of the highly functionalized carbocyclic core of CP-263,114 (phomoidride B), vinyl triflate
13 (phomoidride B) was converted into the olefinic derivative
14 as shown in Scheme 14.
5a
In a study on the stereocontrolled formal synthesis of (±)-platensimycinin, a potent and broad-spectrum Gram-positive antibacterial agent isolated from
Streptomyces platensis, the palladium-catalyzed reduction of a vinyl triflate intermediate gave a good yield of the corresponding alkene by using formic acid as hydrogen source, while tributyltin hydride afforded the alkene in only 37% yield.
62
In a straightforward synthesis of (1-allylcyclohexa-2,5-dienyl)arenes, useful building blocks for the preparation of natural products including amaryllidaceae, strychnos and morphinan alkaloids, the final step consists in palladium-catalyzed formate reduction of the corresponding bis-enol triflate.
63
The palladium-catalyzed formate reduction has been also applied to an asymmetric synthesis of
endo-6-aryl-8-oxabicyclo[3.2.1]oct-3-en-2-one, a natural product from
Ligusticum chuanxing Hort.,
64 to the total synthesis and determination of the absolute configuration of (-)-idesolide, a nitric oxide (NO) production inhibitor isolated from the fruit of
Idesia polycarpa,
65 and to the catalytic asymmetric synthesis of descurainin, isolated from the seeds of
Descurainia sophia (L.) Webb ex Prantl and widely used as Chinese traditional medicine.
66
Palladium-Catalyzed Reduction of Aryl Triflates to Arenes
Alkaloids
The palladium-catalyzed formate reduction of aryl triflates has been extensively applied to the synthesis of aporphines such as 2-fluoro-11-hydroxy-
N-propylnoraporphine,
67 (±)-aporphine,
68 R(-)-
N-alkyl-11-hydroxynoraporphines,
69 (
R)-10-hydroxy- and (
R)-11-hydroxyaporphine,
24b-d,70 11-substituted (
R)-aporphines,
71 (
R)-11-hydroxy-10-methylaporphine
24b,70 esters of
R(-)-
N-alkyl-11-hydroxy-2-methoxynoraporphines,
8b and 2-
O- or 11-
O-substituted
N-alkylnoraporphines
25 in order to evaluate their central serotonergic and dopaminergic effects
in vivo and
in vitro.
Thus, for example, reduction of the aryl triflate
15 was performed in 65% yield in a simple regioselective synthesis of (
R)-11-hydroxyaporphine, a D
1 receptor antagonist, from apomorphine (Scheme 15).
24d
(-)-Galantamine, an alkaloid isolated from the
Amaryllidaceae family, is approved in a number of countries for the treatment of Alzheimer's disease as a selective acetylcholinesterase inhibitor.
Because of the limited supplies and the high cost of its isolation from natural sources, several syntheses of galantamine exploiting a phenolic oxidative coupling of norbelladine-type derivatives have been reported.
In an improved procedure, the extra phenolic hydroxyl group of the intermediate
16 was removed quantitatively by conversion into the triflate
17, followed by the palladium-catalyzed reduction (Scheme 16).
72
During the synthesis of analogues of camptothecin, an anticancer alkaloid isolated from Chinese tree
Camptotheca acuminata, and of camptothecin-related alkaloids, the triflation-reduction sequence has been repeatedly applied to the deoxygenation of phenolic hydroxyl groups of the pentacyclic ring system,
6b,d,e,73 as exemplified in Scheme 17 which outlines the final steps to a close analogue
18 of deoxypumiloside, one of the putative intermediates in the synthesis of camptothecin.
6e
Subjection of the triflates
19 or
20 to conditions for reduction completed two related syntheses of calothrixin B, a carbazole alkaloid isolated from cell extracts of cyanobacterial
Calothrix species endowed with antiprotozoal and anticancer properties, and of a calothrixin B isomer (Scheme 18).
It is worth noting that these transformations could be effected without the need for reprotection of the indole nitrogen atom.
6g,h
Palladium-mediated reduction of both vinyl and aryl triflates has been exploited in the synthesis of a series of thrombin receptor antagonists based on himbacine, a tetracyclic piperidine alkaloid isolated from the bark of the Australian pine tree of Galbulimima species (Scheme 19).
74
Further applications in the area of alkaloids include the synthesis of the ABC ring of safracines,
75 important biogenetic intermediates of saframycins, of analogues of streptonigrin,
76 a 5,8-quinolinedione potent inhibitor of avian myeloblastosis virus reverse transcriptase, of duocarmycin B2,
77 a novel antitumor antibiotic isolated from
Streptomyces sp, of substituted carbazoles and β-carbolines,
78 of eilatin,
79 a polycyclic marine alkaloid, of koumine and koumidine,
80 contained in the Chinese toxic medicinal plant
Gelsemium elegans, of calothrixin B,
81 a natural product with impressive in vitro cytotoxicity, obtained from the cell extract of
Calothrix cyanobacteria, of a calothrixin B isomer,
6h of onychnine,
17a the simplest member of the family of 4-azafluorenone alkaloids, of zoanthenol, the sole member of the zoanthamine alkaloids family possessing an aromatic ring,
82 of (±)-8-oxoerymelanthine, an erythrina alkaloid containing a pyridine ring,
22a of (±)-aurantioclavine, an indole alkaloid characterized by a novel azepino[5.4.3.-
cd)-indole ring,
8a and of simplified analogs of naphthylisoquinoline alkaloids
8c and a SAR study on cassiarin A, a tricyclic alkaloid, isolated from the leaves of
Cassia siamea which shows powerful antimalarial activity against
Plasmodium falciparum in vitro.
17g
Enediynes
In a convergent synthetic route to (+)-dynemicin A, a member of the enediyne family of natural products with potent cytotoxicity against a variety of murine and human tumor cell lines, the key intermediate
21 underwent formate reductive cleavage of the triflate group in 97% yield.
Tributylstannane proved to be slightly less efficient as alternative reducing agent (Scheme 20).
6a,c
The triflation-reduction sequence has been also involved in the synthesis of a hybrid analog of the esperamicin and dynemicin cores.
83
Heterocycles
While the 2-and 3-positions of the indole ring system are relatively easy to functionalise, the preparation of indoles bearing complex functionalities on the benzenoid portion remains a challenge.
In this view, 5-triflyloxyindoles proved to be excellent participants in palladium-catalyzed reactions, including formate reduction.
84 A series of 3,3-bisaryloxindoles has been synthesized and tested as non steroidal human mineralcorticoid receptor (hMR) antagonists.
24e The deoxygenation of hindered bis-triflate
22 was achieved in an acceptable yield, using dppp as bidentate ligand (Scheme 21).
The formate reduction of triflates has been also exploited in a study on the role of the methoxy substituents on the photochromic indolylfulgides
85 and in SAR studies of osthol,
86 a cytotoxic coumarin from
Cnidium monnieri.
The linearly fused furanocoumarins psoralens are of pharmacological interest owing to their ability to cross-link DNA upon irradiation.
Pyridazine analogues of benzopsoralens have been prepared with the aim of improving the stability of the intercalating complex with DNA and convenient substitutions of the pyridazino[4,3-
h]psoralen skeleton through palladium-catalyzed reactions have been explored.
16c Although the Pd/C-catalyzed dehalogenation is a well known method for 3-chloropyridazines, all attempts to apply this procedure to compound
23 using either H
2 or ammonium formate were unsuccessful.
By contrast, the palladium-catalyzed formate reduction of the triflate
24 afforded the desired compound
25 in 80% yield (Scheme 22).
The key step in the synthesis of angelicin (
28), the parent compound of the class of furocoumarins with an angular configuration, utilizing the benzannulation reaction of furylcarbene complexes of chromium, was the palladium-catalyzed reduction of the triflate
26 which was found to proceed smoothly to provide the reduced benzofuran
27 in 89% yield (Scheme 23).
87 In a preliminary communication, the same authors compared the efficiencies of triethylammonium formate and sodium borohydride as reducing agents of a series of aryl triflates and found that, in the case of triflates with electron donating substituents, the reduction with formate was clearly superior, while, in the reduction of 1-naphthyl triflate and of triflates bearing electron withdrawing substituents, the two methods were comparable.
88
4-Aryl-furan-3-ols can be readily converted to the corresponding triflates, which in turn can be reduced with formate to furnish tri-substituted furans.
89
A large class of antimicrobial isoflavonoid phytoalexins contain a substituted pterocarpan ring system.
Pterocarpans such as (±)-homopterocarpin (
31) have been formed directly and efficiently via titanium(IV)-catalyzed cycloadddition of
2H-chromenes with 2-alkoxy-1,4-benzoquinones.
90 In the final step of the synthesis of
31, triflation of
29 followed by a palladium-catalyzed formate reduction of triflate
30 gave (±)-homopterocarpin in 88% overall yield (Scheme 24).
90b
The herbindole and trikentrin indoles comprise a series of structurally related polyalkylated cyclopent[
g]indole natural products with cytotoxic and antibacterial properties, respectively.
During a divergent total synthesis of herbindole B and
cis-trikentrin A, treatment of triflate
32 with ammonium formate under palladium catalysis afforded the deoxygenation intermediate
33 in excellent yield (Scheme 25).
91
Removal of phenol groups via triflates has been further employed in the synthesis of tripodal amidopyridine receptors,
17c tricyclic pyridones,
92 dihydrobenzofurobenzofurans,
93 benzotriazoles,
94 tocopheryl amines and amides,
10 a deoxy derivative of riccardin C, a naturally occurring liver X receptors ligand,
11c a chiral bisdihydrobenzooxaphosphole ligand for rhodium-catalyzed hydrogenations,
12b a spirocyclic piperidine related to biologically active molecules such as MK-677,
15 and diazonamide A, a marine natural product with potent antimitotic activity,
20b in synthetic routes that allow access to either enantiomer of a variety of 2,3-dihydroquinazolinone derivatives,
21f and to cryptophanes,
21g,24i,j in a new alternate synthetic route to remove the 4,5-ether bridge of opioids,
23 and in SAR studies on dopamine autoreceptor antagonists substituted (
S)-phenylpiperidines,
95 inhibitors of the voltage-gated potassium channel Kv1.3 chalcone derivatives of the natural benzofuran product khellinone,
24h and 2-(1,1-dioxo-2H-[1,2,4]benzothiadiazin-3-yl)-1-hydroxynaphthalene derivatives as HCV NS5B polymerase inhibitors.
96
Carbocycles
Alkylphenantrenes (APs) represent a significant class of polycyclic aromatic hydrocarbons useful as synthons for several kind of alkaloids and, in itself, as markers for toxicity.
A combined metalation-Suzuki-Miyaura cross-coupling methodology, involving both direct
ortho (DoM) and remote (DreM) metalation reactions, followed by the palladium-catalyzed hydrogenolysis of the related phenantrols via their triflates, has been adopted to provide short, efficient, and regioselective routes to AP.
97 A similar reductive deoxygenation of phenanthrols was employed in the synthesis of (-)-blestriarene C, a naturally occurring 1,1'-biphenanthrene reported to be active against gram-positive bacteria,
Staphylococcus aureus
, and
Streptococcus mutans.
98 In a general approach to biologically active angucyclines, natural pruducts featuring the benz(a)anthracene ring system, rubiginone B2 was obtained in 96% yield by a chemoselective triflation of hatomarubigin A, followed by the palladium-catalyzed reductive step (Scheme 26).
99
The triflate reduction procedure has been used in a SAR study on 6-naphthalene-2-carboxylate retinoids for RAR transactivation activity.
100 A complete retention of substrate chirality was observed in the deoxygenation of (-)-1,1'-binaphthalene-2,2'-diol via its bis-triflate, while the same reductive conditions were unsuccessful to convert the monotriflates
34 and
35 to the corresponding monosubstituted derivatives (Scheme 27).
7a
Heavily functionalized atropisomeric biphenyl derivatives, useful as liquid crystal dopant or competitive NMDA antagonists, have been synthetized with the use of a Suzuki cross-coupling as the key step, followed by reductive removal of phenolic functional groups.
101 In a versatile route for the synthesis of highly substituted benzenoids, the triflate intermediate was reduced to give trisubstituted benzoate ester in high yield.
102 Alkoxyaromatic compounds, obtained by a regioselective dealkylation of alkyl aryl ethers using niobium(V) pentachloride, were transformed to the corresponding
m-alkoxytoluenes by palladium-catalyzed reduction of their triflates (Scheme 28).
24g
In several SAR studies on 2-aminotetraline derivatives able to interact with 5-HT
1A and/or dopamine D
2-D
3 receptors, with intrinsic activity profiles ranged from full agonists to antagonists and inverse-agonists, the palladium-catalyzed/formic acid reduction of triflate intermediates has been utilized to produce deoxy derivatives from phenolic precursors.
103 (+)-4-Demethoxydaunomycinone, the aglyconic portion of the potent antitumor agent 4-demethoxydaunorubicin, was obtained in five steps through the regioselective formation of the triflate derivative and its palladium-catalyzed reduction (Scheme 29).
104
Benzannulation of a 2,4-dioxygenated aryl Fisher carbene complex, followed by intramolecular Friedel-Crafts cyclization and triflate reduction of the oxygen substituent, provides a synthetic route to a model for the tricyclic core of antitumor antibiotic aureolic acids.
105 In a study of benzobicyclooctanes as novel inhibitors of TNF-a signaling, removal of the aryl hydroxy group was accomplished by the reduction of the corresponding aryl triflate using typical conditions.
106 Use of Pd
2(dba)
3 as catalyst, P(
o-tolyl)
3 as phosphine ligand, and dioxane instead of THF as a solvent were found to be the best reaction conditions to obtain azulene in excellent yield from 2-azulenyl triflate (Scheme 30).
107
Stereoselective synthesis of [5.2]metacyclophane was obtained by means of a [2+2] photocycloaddition and conversion of the phenol intermediate to the deoxygenated product.
108 The synthesis of a dimethylene-bridged clip, belonging to the family of molecules termed molecular tweezers due to their concave-convex topology, was reported.
109 In an improved synthesis of (±)-4,12 dihydroxy[2.2]paracyclophane followed by its enantiomeric resolution by enzymatic method, the (-)-hydroxytriflate derivative was reduced to the known (
S)-(-)-4-hydroxy[2.2]paracyclophane.
110 A cyclotriveratrylene derivative (CVT), involved in the studies of the optical properties of CVTs and in the synthesis of
anti-cryptophane-C, was obtained by the palladium-catalyzed reduction of the bis-triflate precursor, a method claimed to be more appropriate than others to give sizable amounts of deoxygenated CVTs (Scheme 31).
24f Further applications include a concise ring-expansion route to the compact core of platensimycin, a new natural product which was obtained by screening a large collection of South African soil samples using a novel antibiotic assay approach,
111 the synthesis and resolution of two chiral tetraphenylenes, prepared to address the tetraphenylene inversion barrier problem,
7b and the synthesis of analogues of tramadol, a centrally acting opioid analgesic structurally related to codeine and morphine,
112 and of dihalogenated pentiptycenes.
13
Peptides
Palladium-catalyzed reductive deoxygenation of phenols via their triflates was employed in the total synthesis of (
S,S)-isodityrosine, a naturally occurring key structural subunit of numerous biologically active macromolecules.
113 The palladium-catalyzed formate reduction of aryl triflates was employed in the total synthesis of vancomycin and eremomycin aglycons.
21a-c In the reduction step on the intermediate
36, both the aryl triflate on ring 5 and the phenolic allyl protecting group on ring 4 were removed (Scheme 32).
21c
In an investigation on the minimum structure requirements in the aromatic ring moieties for the cytotoxicities of RAs, cyclic hexapeptides originally isolated from the roots of
Rubia akane and
R.
cordifolia showing significant antileukemic and antitumor activities, tyrosine residues in RA-VII and RA-V were deoxygenated, via the corresponding triflates, in yields ranging from 60 to 91%.
114 In a SAR study on the cyclophilin-binding immunosuppressant sanglifehrin A, no reaction occurred in an attempt to remove the phenolic hydroxy group of
38 by palladium-catalyzed reaction of the corresponding triflate using formic acid as hydrogen donor.
The poor reactivity was attributed to the complexation of the palladium catalyst to 1,3-diene systems; indeed, after removal of the double bonds by hydrogenation using palladium on charcoal, the resulting octahydro sanglifehrin A was successfully deoxygenated to
39 by the above method (Scheme 33).
16b
Appendix
Chemical Abstracts Nomenclature (Collective Index Number);
(Registry Number)
Vinyl trifluoromethanesulfonates
vinyl triflate
Cholesta-3,5-diene (747-90-0)
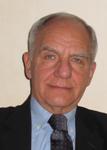 |
Sandro Cacchi graduated in Chemistry at the University of Camerino in 1967.
He then moved to the University of Bologna where he was Assistant Professor.
In 1972 he joined the University "La Sapienza", Rome, where he became Associate Professor in 1983 and, subsequently, full Professor of Organic Chemistry (1986-2010).
Dr.
Cacchi made a contribution early in his career with the development of new, selective synthetic procedures, with particular emphasis on the chemistry of molecules of biological interest.
In the late seventies, he moved to the exciting new field of palladium chemistry and the utilization of palladium catalysis in organic synthesis became a major goal that he pursued in many ways, with the search for new and selective methodologies being a major thrust even in this area.
|
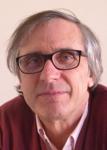 |
Enrico Morera received his degree in Pharmacy in 1974 from University of Rome (Italy).
After a fellowship from the Ministry of Education, he was appointed Researcher and then Associate Professor in Medicinal Chemistry at the Faculty of Pharmacy of the University of Rome 'Sapienza'.
The research activity was at first devoted to the study of new synthetic methods and their application to the synthesis of steroid, heterocycle and aminoacid derivatives.
More recently his research has centered around the field of non-proteinogenic α-aminoacid, and on biological systems such as endocannabinoid system, transient receptor potential channels (TRPs) and on connection between them. |
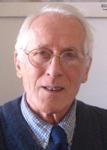 |
Giorgio Ortar was born in Gorizia in 1943.
He graduated in Chemistry at the University of Rome 'Sapienza' in 1967.
In 1972 he was appointed researcher of Italian National Council of Researches (CNR) at the Centro di Chimica del Farmaco of Rome and held this position until 1987.
From 1987 he is Associate Professor of Medicinal Chemistry at the Faculty of Pharmacy of the University of Rome 'Sapienza' His scientific activity has been concerned initially with studies on oxidation, solvolysis, and rearrangement reactions of steroidal substrates.
Then, he devoted himself for many years to studies on palladium-catalyzed reactions with a number of applications to molecules of medicinal interest.
In the last seven years his researches have been mainly focused on endocannabinoid system and on transient receptor potential (TRP) superfamily of ion channels. |
Copyright © 1921-, Organic Syntheses, Inc. All Rights Reserved