Org. Synth. 2012, 89, 210-219
DOI: 10.15227/orgsyn.089.0210
Synthesis of Trifluoromethyl Ketones from Carboxylic Acids: 4-(3,4-Dibromophenyl)-1,1,1-trifluoro-4-methylpentan-2-one
[]
Submitted by Jonathan T. Reeves
1, Zhulin Tan, Daniel R. Fandrick,
Jinhua J. Song, Nathan K. Yee, and Chris H. Senanayake.
Checked by David Hughes
1. Procedure
A. 3-(3,4-Dibromophenyl)-3-methylbutanoic acid (3). A 250-mL, oven-dried three-necked round-bottomed flask, equipped with a 3-cm oval PTFE-coated magnetic stirring bar, is charged with aluminum chloride (13.4 g, 100 mmol, 2.0 equiv) (Note 1). The flask is fitted with a gas inlet adapter connected to a nitrogen line and a gas bubbler. The other two necks are capped with rubber septa; a thermocouple probe is inserted through one of the septa (Note 2). Dichloromethane (8 mL) and 1,2-dibromobenzene (8.0 mL, 15.7 g, 67 mmol, 1.3 equiv) are added via pipette and stirring is initiated. To the resultant slurry is charged a solution of 3,3-dimethylacrylic acid (5.00 g, 50 mmol, 1.00 equiv) in dichloromethane (10 mL) via syringe over 10 min, maintaining the internal temperature = 32 °C. The reaction mixture is stirred at room temperature for 2.5 h (Note 3). A 1-L Erlenmeyer flask equipped with a 5 cm flat stir bar and a thermocouple probe (Note 2) is charged with water (150 mL) followed by concentrated hydrochloric acid (150 mL) and cooled to 2 °C in an ice-water bath. The Friedel-Crafts reaction mixture is transferred portion-wise via a 9-inch disposable glass pipette into the stirred, cold aqueous HCl solution over 5 min (Note 4). Ethyl acetate (200 mL) is used to rinse residues from the reaction flask into the aqueous HCl. The biphasic mixture is then transferred to a 1-L separatory funnel and the lower aqueous phase is discarded. The organic phase is washed with water (100 mL), then brine (50 mL). The organic phase is filtered through a bed of sodium sulfate (30 g) in a medium porosity sintered glass funnel into a 500-mL round-bottomed flask, using ethyl acetate (2 x 50 mL) to rinse the filter cake. The solution is concentrated by rotary evaporation (50 °C, 20 mmHg) to a yellow oil (21 g, (Note 5)). The flask is charged with heptane (50 mL) and warmed to 60 &Degree;C using a water bath to complete dissolution (Note 6). The solution is allowed to cool to room temperature over about 2 h, during which time crystallization occurs. The slurry is further cooled to 0-5 °C by placement in a refrigerator overnight. The slurry is filtered through a medium porosity sintered glass funnel, washed with heptane (2 x 20 mL) pre-cooled to 0-5 °C, and then air-dried to constant weight to provide 3-(3,4-dibromophenyl)-3-methylbutanoic acid 3 as an off-white solid (12.1-12.8 g, 72-76% yield) (Note 7).
B. 4-(3,4-Dibromophenyl)-1,1,1-trifluoro-4-methylpentan-2-one (4). A 250-mL three-necked, round-bottomed flask, equipped with a 3-cm oval PTFE-coated magnetic stirring bar and a reflux condenser fitted at the top with a gas inlet adapter connected to a nitrogen line and a gas bubbler, is charged with 3-(3,4-dibromophenyl)-3-methylbutanoic acid (3) (10.0 g, 29.7 mmol, 1.00 equiv) and toluene (70 mL) (Note 8). The other two necks are capped with rubber septa; a thermocouple probe is inserted through one of the septa (Note 2). To the resulting stirred slurry is charged trifluoroacetic anhydride (18.5 mL, 4.50 equiv) (Note 9). Then pyridine (14.5 mL, 6.0 equiv) is added dropwise (exothermic) via syringe over 5 min keeping the internal temperature of the reaction mixture = 40 °C. The flask is heated using a heating mantle for 4 h, maintaining the internal temperature at 60-65 °C (Note 10). The reaction mixture is cooled to 2 °C in an ice-water bath. The reflux condenser is replaced with a 125-mL addition funnel through which is charged water (50 mL) to the reaction mixture over 5 min, maintaining the internal temperature of the reaction mixture < 40 °C (Note 11). The ice-water bath is removed and replaced with a heating mantle and the mixture is heated for 1 h, maintaining an internal temperature of 57-60 °C (Note 12). The reaction mixture is cooled to room temperature and transferred to a 500-mL separatory funnel using hexanes (150 mL) and water (100 mL). The layers are separated and the aq. layer is back extracted with 100 mL of 2:1 hexanes:toluene. The combined organic layers are washed with water (100 mL), then filtered through a bed of sodium sulfate (30 g) in a medium porosity sintered glass funnel into a 500-mL round-bottomed flask, using hexanes (2 x 50 mL) to rinse the filter cake. The filtrate is concentrated by rotary evaporation (50 °C, 20 mmHg) to an orange oil (13 g) (Note 13). The oil is purified by flash column chromatography (Note 14) to provide 4-(3,4-dibromophenyl)-1,1,1-trifluoro-4-methylpentan-2-one (4) as a light yellow oil (9.04-9.68 g, 78-84% yield) (Note 15).
2. Notes
1.
The following reagents and solvents in step A were used as received:
aluminum chloride (Acros, 99%, powder), 1,2-dibromobenzene (98%, Sigma-Aldrich), 3,3-dimethylacrylic acid (Sigma-Aldrich, 97%), dichloromethane (Fisher, ACS reagent, 99.5%), ethyl acetate (Fisher, ACS reagent, 99%), 37% HCl (Fisher), and heptane (Sigma Aldrich, Chromasolv, 99%).
Deionized water was used throughout.
2.
The internal temperature was monitored using a J-Kem Gemini digital thermometer with a Teflon-coated T-Type thermocouple probe (12-inch length, 1/8 inch outer diameter, temperature range -200 to +250 &Degree;C).
3.
The checker monitored the reaction by
1H NMR as follows. A
0.1 mL aliquot of the reaction mixture was quenched into
1 mL CDCl
3 and
1 mL 6N HCl. The CDCl
3 layer was analyzed by NMR. Diagnostic resonances were δ 5.7 (1 H), 2.2 (3 H), and 2.0 (3 H) for
2 and 2.63 (2 H) and 1.43 (6 H) for
3. At the 90 min time point, approx 4% unreacted
2 remained. The submitters monitored both reactions using reverse phase HPLC employing the following conditions: column: TSK-gel SuperODS column (ID 4.6 mm, length 5.0 cm, particle size 2 μm); detection at 220 nm; mobile phase of 10% ramping to 90% MeCN in water (with 0.05 volume% TFA in both phases) over 3.5 min, then hold at 90% MeCN in water for 1.5 min; flow rate: 2.0 mL/min. The starting materials, products and solvents had the following retention times:
1 (3.1 min);
2 (1.2 min);
3 (2.9 min);
4 (3.7 min); toluene (2.7 min). TLC may also be used. The starting materials and products have the following R
f values in 60:40 hexanes/EtOAc:
1 (0.9);
2 (0.7);
3 (0.35);
4 (0.9). In 95:5 hexanes/MTBE,
4 has an R
f value of 0.5.
4.
The addition of the Friedel-Crafts reaction mixture into the aqueous HCl is exothermic. The temperature at the end of transfer was 20 °C.
5.
The composition of the crude material by
1H NMR (by wt%) was approx. 83% product
3, 11%
1,2-dibromobenzene, 5 % EtOAc, and 1% unreacted
2 (3 mol %).
6.
In most experiments the yellow oil crystallized prior to addition of heptane. Heating the heptane mixture to 60 °C effects dissolution of the solid, therefore a true recrystallization is achieved.
7.
3-(3,4-Dibromophenyl)-3-methylbutanoic acid 3 has the following physical and spectroscopic properties: off-white solid; mp 89-91 °C; IR (neat) 2966, 1710, 1648, 1473, 1377, 1250, 1166, 1011 cm
-1; MS
m/z (relative intensity), 320.9 (21), 318.8 (42), 316.8 (21) [M-OH]; 278.9 (49), 276.8 (100), 274.8 (50) [M-CH
2CO
2H]; 215.0 (43), 141.0 (64), 127.0 (59), 118.0 (54), 102.0 (63);
1H NMR
pdf(CDCl
3, 400 MHz) δ: 1.43 (s, 6 H), 2.63 (s, 2 H), 7.16 (dd,
J = 8.5, 2.3 Hz, 1 H), 7.54 (d,
J = 8.4 Hz, 1 H), 7.60 (d,
J = 2.3 Hz, 1 H), 11.1 (broad s, 1 H);
13C NMR
pdf(CDCl
3, 100 MHz) δ: 29.0, 37.0, 47.7, 122.4, 124.9, 126.7, 131.3, 133.5, 149.3, 177.4; Anal. Calcd. For C
11H
12Br
2O
2: C, 39.32; H, 3.60. Found: C, 39.49; H, 3.29; HPLC area % purity: 95 % (HPLC method: column, fused-core C-18, 4.6x100 mm, 2.7 µm particle size; gradient elution using a mobile phase of 90% aq. 0.1% H
3PO
4/10% MeCN to 10% aq. 0.1% H
3PO
4/90% MeCN over 6 min, holding the latter for 2 min; flow rate
1.8 mL per min; temperature 40 &Degree;C, detection at 210 nm; t
R for
3 was 4.8 min.)
8.
The following reagents and solvents in step B were used as received: toluene (Sigma-Aldrich, Chromasolv, 99.9%), trifluoroacetic anhydride (Sigma-Aldrich, Reagent Plus, = 99%), pyridine (Sigma-Aldrich, 99%), hexanes (Fisher, ACS reagent, >98.5%),
methyl t-butyl ether (Sigma-Aldrich), and silica gel (Fisher, 230-400 mesh, 60 Å).
9.
The addition of TFAA is slightly endothermic. The temperature of the reaction mixture generally decreases by 2-4 °C after the TFAA is added.
10.
The submitters monitored the reaction for consumption of starting acid
3 by HPLC and TLC
(Note 3). The checker monitored the reaction by
1H NMR by quenching a
0.1 mL aliquot of the reaction mixture in
1 mL CDCl
3 and
1 mL water, concentrating the CDCl3 to remove residual toluene, then redissolving in
CDCl3. The reaction samples at the 4 h time point had no detectable starting material (monitoring resonances at δ 2.63 and 1.43). Product
4 was the major species present.
11.
The addition of water is highly exothermic, particularly for the first few mL. The addition is also accompanied by the evolution of CO
2 gas.
12.
Heating the reaction mixture at 60 °C for 1 hour drives the decarboxylation to completion.
13.
The crude material by
1H NMR was approx. 87 wt % product, 13 wt % toluene.
14.
The checker used flash chromatography to purify product as follows: 150 g
silica gel with an eluent of 300 mL 2% MTBE/hexanes,
300 mL 3% MTBE/hexanes,
600 mL MTBE/hexanes;
50 mL fractions. Product eluting in fractions 8-21 were combined and concentrated by rotary evaporation (40 °C, 20 mmHg), then held under vacuum (20 mmHg) for 14 h at room temperature to afford product as a yellow oil. Submitters used a Combi-Flash apparatus using a 150 g silica gel column using
hexanes/methyl t-butyl ether (100:0 to 95:5 v/v) as eluent, collecting
25 mL fractions at a flow rate of 85 mL/min, and the UV detector was set at 220 nm. Residual toluene eluted first (2-4 min) with 100% hexanes. The eluent was then ramped over 13 min from 0 to 5% MTBE in hexanes. The product began eluting at 10 min and elution was complete after 26 min (50 fractions of
25 mL were required to collect the product.
15.
4-(3,4-Dibromophenyl)-1,1,1-trifluoro-4-methylpentan-2-one 4 has the following physical and spectroscopic properties: light yellow oil; IR (neat) 2971, 1765, 1469, 1380, 1285, 1200, 1149, 1025 cm
-1; GC-MS m/z (relative intensity), 390 (31), 388 (62), 386 (31), [M
+], 279 (84), 277 (100), 275 (86), [M - CH
2COCF
3], 251 (20), 249 (38), 247 (20), 115 (45);
1H NMR
pdf(CDCl
3, 400 MHz) δ: 1.46 (s, 6 H), 3.05 (s, 2 H), 7.13 (dd,
J = 8.5, 2.4 Hz, 1 H), 7.55 (d,
J = 8.5 Hz, 1 H), 7.59 (d,
J = 2.4 Hz, 1 H);
13C NMR
pdf(CDCl
3, 100 MHz) δ: 28.8, 36.8, 48.5, 115.3 (q,
J = 293 Hz, 1 C), 122.7, 125.2, 125.9, 131.1, 133.7, 148.5, 189.1 (q,
J = 35 Hz, 1 C); Purity by GC: 97% (t
R = 11.7 min; conditions: Agilent DB35MS column; 30 m x 0.25 mm; initial temp 60 °C, ramp at 20 °C/min to 280 °C, hold 15 min); two peaks were present by reverse phase HPLC (conditions outlined in
(Note 7)): t
R 5.3 min (30%, ketone hydrate of
4) and 6.2 min (70%, ketone product
4).
Handling and Disposal of Hazardous Chemicals
The procedures in this article are intended for use only by persons with prior training in experimental organic chemistry. All hazardous materials should be handled using the standard procedures for work with chemicals described in references such as "Prudent Practices in the Laboratory" (The National Academies Press, Washington, D.C., 2011 www.nap.edu). All chemical waste should be disposed of in accordance with local regulations. For general guidelines for the management of chemical waste, see Chapter 8 of Prudent Practices.
These procedures must be conducted at one's own risk. Organic Syntheses, Inc., its Editors, and its Board of Directors do not warrant or guarantee the safety of individuals using these procedures and hereby disclaim any liability for any injuries or damages claimed to have resulted from or related in any way to the procedures herein.
3. Discussion
Trifluoromethyl ketones are useful intermediates for the introduction of the trifluoromethyl group into organic molecules.
2 While several methods for the synthesis of
trifluoromethyl ketones have been reported, the procedure reported by Zard and co-workers is attractive in terms of simplicity.
3 This pioneering work involves treating primary
(α-CH2) acid chlorides at room temperature with
trifluoroacetic anhydride and pyridine in CH2Cl2 for 0.5 - 8 hours, followed by hydrolysis/decarboxylation on addition of water. The application of the Zard procedure to more hindered primary acid chlorides, however, gives the trifluoromethyl ketone products in low conversions and yields, even after extended reaction times. It was found that by changing the solvent from
CH2Cl2 to toluene and increasing the reaction temperature to 60 °C, more hindered substrates underwent full conversion and provided products in good yields. Furthermore, this process proved to be effective for the direct conversion of carboxylic acids to trifluoromethyl ketones (Table 1).
4 This avoids the need for conversion of the carboxylic acid to the corresponding acid chloride prior to trifluoromethyl ketone formation. Secondary (α-branched) carboxylic acids may also be converted to trifluoromethyl ketones if the reaction temperature is further increased to 100 °C. We have found this procedure is particularly well suited to
3,3-dimethyl-3-arylpropionic acid substrates, such as acid
3, (entry 1) which contain aryl bromide functionality that is not stable to alternative methods employing strong bases. The starting acid
3 is conveniently prepared by a Friedel-Crafts reaction of
3,3-dimethylacrylic acid 2 with
1,2-dibromobenzene 1.
Table 1.Examples of Direct Conversion of Carboxylic Acids to Trifluoromethyl Ketones
The proposed mechanism of the reaction involves a-trifluoroacetylation of a pyridinium enolate.
3 The pyridinium enolate may be derived from an acid chloride precursor in the case of Zard's reaction conditions
3 or a mixed anhydride of the starting
carboxylic acid and trifluoroacetic acid in the present case (Scheme 1).
4
Scheme 1.Proposed Mechanism for Acid to Trifluoromethyl Ketone Conversion
Appendix
Chemical Abstracts Nomenclature (Collective Index Number);
(Registry Number)
; (Registry Number)
Aluminum Chloride; (7446-70-0)
1,2-Dibromobenzene; (583-53-9)
3,3-Dimethylacrylic acid: 2-Butenoic acid, 3-methyl- ; (541-47-9)
Trifluoroacetic anhydride;
(407-25-0)
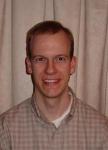 |
Jonathan T. Reeves was born in 1975 in Michigan, USA. He received a B.S. degree in chemistry from Hope College, Holland, MI in 1997. He then received a Ph.D. in organic chemistry under the guidance of Professor Peter Wipf at the University of Pittsburgh in 2002. After two years as an NIH postdoctoral fellow at Indiana University with Professor David R. Williams, he joined the Chemical Development department at Boehringer Ingelheim Pharmaceuticals, Inc., in Ridgefield, CT, where he is currently a Principal Scientist. His research interests include organometallic, heterocyclic and asymmetric methodology development.
|
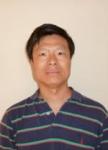 |
Zhulin Tan received a B.S. degree in chemistry from Sichuan University in Chengdu, China (1988). He then joined Sichuan Chemical Engineering Institute as a research associate in Chengdu, China. After a brief stay at the University of Delaware, he moved to Worcester Polytechnic Institute in 1996 and obtained his M.S. in organic chemistry in 1999 under the guidance of Professor Stephen Weininger. He then joined the Department of Chemical Development at Boehringer Ingelheim Pharmaceuticals in Ridgefield, CT, where he is currently a Senior Research Associate. His research interests include heterocyle synthesis and organocatalysis using N-heterocyclic carbenes.
|
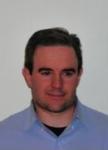 |
Daniel R. Fandrick received his B.S. degree in chemistry from the University of California, San Diego under the guidance of Professor Joseph M. O'Conner. In 2006, Daniel received his PhD degree in organic chemistry at Stanford University under the mentorship of Professor Barry M. Trost. His graduate studies focused on the development of the dynamic kinetic asymmetric transformations of vinyl aziridines and allenes with applications to total synthesis. After graduation, he joined the chemical development group at Boehringer-Ingelheim. His main interests are in the development of transition metal catalyzed and sustainable methodologies to provide efficient access to pharmaceutically useful scaffolds.
|
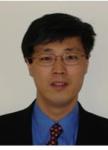 |
Dr. Jinhua Jeff Song received his undergraduate education at Nankai University in Tianjin, China (1992). After a brief stay at Rice University in Houston, TX, he moved to the Massachusetts Institute of Technology in 1993 and obtained his Ph.D. degree in 1998 under the supervision of Prof. Satoru Masamune. Subsequently, he joined the Department of Chemical Development at Boehringer Ingelheim Pharmaceuticals, where he is currently Senior Associate Director. His research areas encompass natural product synthesis, asymmetric synthesis of chiral biologically active compounds, efficient methodologies for heterocycle synthesis, and novel N-heterocyclic carbene-catalyzed reactions. Some of his work also received media attention and has been highlighted in the Chemical and Engineering News. Additionally, Dr.
Song holds >15 patents on efficient synthesis of pharmaceutical agents.
|
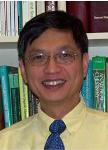 |
Dr. Nathan Yee received his B.S. degree from the University of New York at Stony Brook in 1985, and then moved to the University of Illinois at Urbana-Champaign and obtained his Ph.D. degree in 1991 under the supervision of Prof. Robert Coates. He was a postdoctoral fellow at Yale University with Prof. Frederick Ziegler in 1991-1992. In 1992 he joined Boehringer Ingelheim Pharmaceuticals, Inc. as a Senior Scientist in Chemical Development, and he is currently Director of Chemical Development. He has been leading multi-disciplined teams engaged in Process Research, Analytical Research, and Solid State Physicochemical Characterization. His research interests include the development of new synthetic methodologies and their applications to chemical processes for drug substance manufacturing.
|
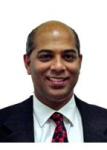 |
Dr. Chris H. Senanayake was born in Sri Lanka and received a BS degree (First Class) in Sri Lanka. He completed his MS at Bowling Green State University and his Ph.D. under the guidance of Professor James H. Rigby at Wayne State University in 1987. After postdoctoral studies with Professor Carl R. Johnson he joined the Department of Process Development at Dow Chemical Company in 1989. Currently, he is the Vice President of Chemical Development for Boehringer Ingelheim Pharmaceuticals and leading a group of highly talented scientists, engineers, and administrative staff located in Ridgefield, CT. Dr. Senanayake's research interests focus on the development of new asymmetric methods for the synthesis of bioactive molecules and heterocycles and on catalytic, enzymatic, and mechanistic studies.
|
Copyright © 1921-, Organic Syntheses, Inc. All Rights Reserved