Org. Synth. 2012, 89, 350-373
DOI: 10.15227/orgsyn.089.0350
Discussion Addendum for:
[Synthesis of 1,2:4,5-Di-o-isopropylidene-D-erythro-2,3-hexodiulo-2,6-pyranose.
A Highly Enantioselective Ketone Catalyst for Epoxidation/Asymmetric Epoxidation of trans-β-Methylstyrene and 1-Phenylcyclohexene using a D-Fructose-derived Ketone: (R,R)-trans-β-Methylstyrene Oxide and (R,R)-1-Phenylcyclohexene Oxide]
Submitted by Thomas A. Ramirez, O. Andrea Wong, and Yian Shi
*1.
Discussion
Prior to and subsequent to the original reports in
Organic Syntheses, we have reported a number of carbohydrate-derived ketone epoxidation catalysts
2 including
1-
4 (Figure 1).
Epoxidation of olefins catalyzed by these ketones has become a valuable tool for the synthetic organic chemist.
2
Figure 1. Four Generations of Ketone Catalysts
Generations of Catalysts:
Ketone 1:
The synthesis and use of ketone
1 was reported in the original
Organic Syntheses articles.
Ketone
1 is an effective catalyst for the epoxidation of a variety of
trans- and tri-substituted olefins,
3 vinylsilanes,
4 hydroxy alkenes,
5 dienes,
6 enynes,
7 enol ethers, and enol esters.
8,9 A procedure for the preparation of
ent-ketone
1 from L-sorbose has also been developed.
3b,10 Ketone
1 and
ent-ketone
1 have been successfully used in various synthetic applications.
2h-k
Ketone 2:
Ketone
2 has proven to be a more reactive analogue of ketone
1, particularly in the reaction of electron-deficient olefins.
It is derived from ketone
1 through a selective deketalization followed by acetylation.
11
Ketones 3:
Ketones
3 mediate the highly stereoselective epoxidation of conjugated
cis-, terminal, tri-substituted, and some tetra-substituted double bonds.
12-21
While ketone
3a is highly effective,
3c-
3f were developed due to their streamlined syntheses.
13 Ketones
3a and
3f have also found efficacy in the epoxidation of non-conjugated, amphiphilic
cis-olefins.
22
Ketones 4:
Ketone
4a has extended the capability of the epoxidation system to geminally-disubstituted terminal olefins and also works well in the epoxidation of some
cis- and tri-substituted olefins.
23 Ketone
4b has proven effective in the reaction of
trans- and tri-substituted double bonds.
23
Ketone
1,
ent-ketone
1,
3c, and
3d are now commercially available.
Our group website now features "Guidelines for Asymmetric Epoxidation"
24 which includes instructive illustrations and tips for running the epoxidation reactions, and the document addresses frequently asked questions.
Synthetic Applications:
Previously published reviews provide a detailed discussion of the development of the catalysts and their respective substrate scopes.
2b-e, 2g-k This update will focus primarily on the wealth of synthetic applications of ketone
1 and
ent-ketone
1.
Ketone 1:
The epoxidation with ketone
1 is practical and has been used in undergraduate teaching laboratories,
25 academic research laboratories,
2b-e,g-k and in industry.
26 Along with other proven asymmetric epoxidation systems, ketone
1 has validated the use of stereoselective epoxidation in endgame approaches as well as those invoking the epoxide's manipulability.
In addition, the ketone-catalyzed epoxidation is a process that can be used for discriminant or indiscriminant (poly-) epoxidation which can be further utilized in the rapid construction of complex molecules via cascade cyclization reactions.
Figure 2. Examples of Target Molecules Synthesized with Epoxidation Catalyzed by Ketone 1 or ent-Ketone 1
Some examples of the use of ketone
1 in the selective installation of the epoxide functional group in biologically active molecules are shown in Figure 2.
Altmann and coworkers used
ent-ketone
1 for the epoxidization of an unsaturated macrolide to afford an epothilone analogue as a single isomer.
27 Ready and coworkers utilized ketone
1 for a highly discriminant epoxidation in their synthesis of (+)-nigellamine A
2.
28 Moher,
29 Kotake,
30 and others
31,32 have used ketone
1 to stereoselectively install epoxides in the side chains of target molecules.
The ketone-catalyzed epoxidation has found use in industry.
26 A team at DSM Pharma Chemical obtained lactone
7 in 63% overall yield and 88% ee when crude α,δ-unsaturated carboxylate was subjected to the reaction conditions (Scheme 1).
26 The intermediate epoxide was opened by an intramolecular attack by the carboxylate anion.
22,26 After the product was crystallized from heptane, 59 lbs of lactone were obtained.
Scheme 1. Industrial Scale Synthesis of Lactone 7 via Epoxidation/Epoxide Opening
Sudalai and coworkers have used a similar process in their synthesis of (+)-L-733,060 (
10), an antitumor agent with activity against retinoblastoma (Scheme 2).
33 The γ,δ-unsaturated carboxylate
8 was stereoselectively epoxidized and then underwent
in situ lactonization.
Scheme 2. Synthesis of (+)-L-733,060
Myers and coworkers developed a method to synthesize
trans-1,2-diol derivatives
12 using ketone
1-catalyzed epoxidation of cyclic
E and acyclic
E and
Z silyl enol ethers followed by reduction mediated by an internal hydride delivery (Scheme 3).
34,35
Scheme 3. Epoxidation/Reduction For 1,2-Diols
McDonald and coworkers used ketone
1-catalyzed epoxidation to access potentially antineoplastic 1-deoxy-5-hydroxysphingosine analogue
16 (Scheme 4).
36 Lewis acid catalyzed cyclization led to cyclic carbonate
15.
The resulting alcohol was then activated and displaced by an azide to yield the 2-amino-3,5-diol motif.
The C-5 epimer of
16 was also synthesized when
ent-13 was used as the starting template.
Scheme 4.
Synthesis of 1-Deoxy-5-Hydroxysphingosine Analogue
Scheme 5.
Synthesis of (-)-8-Deoxyserratinine
Yang and coworkers recently reported the total synthesis of (-)-8-deoxyserratinine (
19) (Scheme 5).
37 Ketone
1 allowed them to overcome the substrate-induced facial bias in the epoxidation of
17 which afforded
18 as the sole isomer.
Huo and coworkers recently synthesized (+)-neroplofurol (
23) in two operations and 60% overall yield from the naturally (and commercially) available (+)-nerolidol (Scheme 6).
38 Upon epoxidation of
21, cyclization took place
in situ.
Scheme 6.
Synthesis of (+)-Neroplofurol (23)
Wiemer and coworkers have also investigated the use of cascade cyclizations in the syntheses of schweinfurthins.
39 A ketone
1-catalyzed highly site- and stereo-selective epoxidation followed by a Lewis acid catalyzed cyclization allowed them to synthesize schweinfurthin B (
27)
39 (Scheme 7) and a diverse array of derivatives.
Scheme 7.
Synthesis of Schweinfurthin B (27) via Epoxidation/Cascade Cyclization
In an effort to confirm the absolute stereochemistry of glabrescol (
30), Corey and coworkers obtained the poly-tetrahydrofuran via the tetra-epoxidation of tetra-ene
28 followed by a CSA-mediated cyclization (Scheme 8).
40,41
Scheme 8.
Synthesis of Glabrescol (30)
In 2010, (+)-omaezakianol was obtained by Xiong, Busch, and Corey via penta-epoxidation of
31 followed by cascade cyclization and dehalogenation (Scheme 9).
42
Scheme 9.
Synthesis of (+)-Omaezakianol (34)
In 2009, Morimoto and coworkers accomplished a synthesis of (+)-omaezakianol (
34) using a convergent approach (Scheme 10).
43 Ketone
1 promoted a
bis-epoxidation of
35 in high yield and diastereoselectivity.
Compound
37 was assembled, in part, by an
ent-ketone
1 catalyzed epoxidation.
Epoxide
36 and
37 were cross-coupled and a cascade cyclization along with some additional steps afforded the target product.
Scheme 10.
Synthesis of (+)-Omaezakianol (34)
Scheme 11.
Polyepoxide Cyclization
McDonald and coworkers have reported on a series of biomimetic cascade cyclizations of poly-epoxides to access complex polycyclic ethers.
An example is shown in Scheme 11.
44a The Sharpless methodology was used to epoxidize the allylic alcohol and ketone
1 facilitated the tris-epoxidation of
40.
The carbamate was used to trigger the cascade cyclization under Lewis-acidic conditions to afford fused tetracycle
42.
44
Jamison and coworkers have shown that poly-vinyl silane
46 can be poly-epoxidized to afford
47, which was converted to a ladder polyether under basic conditions in methanol.
The trimethylsilyl groups directed the 6-
endo attack and then "disappeared" (Scheme 12).
45
Scheme 12.
Stereoselective Tris-Epoxidation and Subsequent TMS-Directed Cyclization
Scheme 13.
Stereoselective Tris-Epoxidation and Subsequent Water-Promoted Endo-Cyclization
In their subsequent studies, Jamison and coworkers have reported that water can promote the highly regioselective 6-
endo cyclization of tethered di-and tri-epoxides thus bypassing the need for the TMS-directing groups (Scheme 13).
46 Additionally, the water allows the 6-
endo cyclization to override regioselective bias induced by methyl groups on the epoxides.
46c They have also shown that the
1,3-dioxane group can be a suitable template for
endo-selective cyclizations of epoxides.
46f,47-49
Marshall and coworkers assembled the C17-C32 fragment of the antibiotic ionomycin (Scheme 14).
50 Sharpless epoxidation installed the peripheral epoxides in
53 and ketone
1 catalyzed the epoxidation of the internal double bond, resulting in
54.
50-52 The zinc debrominates
55 and facilitates the cascade cyclization.
Scheme 14.
Synthesis of C17-C32 Fragment of Ionomycin
Scheme 15.
Synthesis of (+)-Aurilol (63)
In 2005, Morimoto and coworkers reported the first total synthesis of (+)-aurilol (
63) (Scheme 15).
53 A series of epoxidations catalyzed by ketone
1 and
ent-ketone
1 allowed them to construct the cyclic ethers in a regio- and stereo-controlled fashion.
McDonald and coworkers synthesized
ent-abudinol B (
69) utilizing epoxidation/cascade cyclization (Scheme 16).
54 Bis-epoxidation of
64 with ketone
1 resulted in high diastereoselectivity in the formation of
65.
A second iteration of
bis-epoxidation resulted in
68 which formed
69 upon treatment with TMSOTf.
They had reported an earlier synthesis of the same molecule which also employed ketone
1-catalyzed epoxidation.
54d
Scheme 16.
Synthesis of ent-Abudinol B (69)
Uenishi and coworkers stereoselectively constructed linked tetrahydrofurans with a Pd(II)-catalyzed cascade cyclization (Scheme 17).
55 The metal serves to activate the epoxide while controlling the regioselectivity and also to catalyze and transfer the chirality of the displaced allylic alcohol in the S
N2'-type reaction.
Scheme 17.
Pd(II)-Catalyzed Formation of Linked Tetrahydrofurans
Micalizio and coworkers synthesized a tetracyclic fragment
79 of pectenotoxin-2 (Scheme 18).
56 Ent-Ketone
1/H
2O
2 epoxidized the double bond in
75 with excellent diastereoselectivity and subsequent deprotection of the TES-protected alcohol led to formation of THF
77.
Deprotection and oxidation of the TBS-protected alcohol led to a domino condensation/cyclization to form
78.
Scheme 18.
Synthetic Work Toward Pectenotoxin-2
Lee, Gagné, and coworkers have shown that allenes can also initiate these cascade reactions.
57 In the example shown in Scheme 19, a tandem of Sharpless and ketone
1-catalyzed epoxidations set the stereochemistry in
81, and upon treatment with Au(I), the allene reacts with the proximal epoxide to set off the cascade.
The product was obtained in good yield and high d.r.
Scheme 19.
Allene-Initiated Cascade Cyclization
The previous section has given a snapshot of the synthetic utility of ketone
1 and
ent-ketone
1.
The use of this methodology in synthesis
58 and dynamic method developments
58-61 are anticipated to undergo continued growth.
Ketone 2:
Ketone
2 has recently begun to receive more attention in organic synthesis.
Suzuki and coworkers completed the first synthesis of
ent-seragakinone A (
87) (Scheme 20) and confirmed its absolute stereochemistry.
62 Substrate-controlled epoxidation of
84 resulted in low diastereoselectivity, however
ent-ketone
2 induced good diastereoselectivity (8.2:1 d.r.).
Acid-promoted hydration of the epoxide and subsequent conjugate addition provide the THF in compound
86.
This provided the tetracyclic core which was further elaborated to
87.
Scheme 20.
Synthesis of ent-Seragakinone A (87)
Hamada and coworkers have used ketone
2 to synthesize precursors to valuable compounds such as angelmarin, (+)-marmesin, (-)-(3'R)-decursinol, and (+)-lomatin.
63 Synthetic work toward angelmarin, a chemical that displays activity against PANC-1 cancer cells, is shown in Scheme 21.
63 Epoxidation with ketone
2 led to the formation of
89 in 88% yield and 97% e.e.
Employing a two-step process for the removal of the TBS group and cyclization at low temperatures allows for highly selective (and complementary) dihydrobenzopyran formation.
63 Kan and coworkers have used ketone
2-catalyzed epoxidation to synthesize epimeric epigallocatechin gallates.
64
Scheme 21.
Synthesis of Angelmarin (90) using Ketone 2
Yadav and coworkers have achieved an expedient formal synthesis of diastereomeric Hagen's gland lactones using ketone
2.
65 Bruner and coworkers epoxidized
trans-ethyl cinnamates and saponified the esters to study the products' ability to inhibit
SgTAM, an MIO-based aminomutase.
66
Scheme 22.
Synthesis of Lactodehydrothyrsiferol (94)
Floreancig and coworkers recently accomplished the
bis-epoxidation of
91 in high stereoselectivity using
ent-ketone
1 and ketone
2 (Scheme 22).
Ent-ketone
1 catalyzed the epoxidation of the more reactive double bond, and upon completion, ketone
2 was added to the pot to facilitate epoxidation of the less reactive site.
They then further elaborated
92 to lactodehydrothyrsiferol (
94).
67
Shi and coworkers recently obtained 120 g of virtually enantiopure (+)-ambrisentan (
97) without the need for column chromatography (Scheme 23).
68 (+)-Ambrisentan, an endothelin-1 receptor antagonist, is currently used to treat hypertension.
Ketone
2-catalyzed epoxidation afforded
96 in 90% conversion and 85% ee.
Compound
97 was further enriched via precipitation and filtration of the racemate.
Scheme 23. Synthesis of (S)-Ambrisentan
Ketones 3:
Ketones
3 have been used in the asymmetric epoxidation/rearrangement of benzylidene cyclopropanes to synthetically valuable cyclobutanones and lactones and in the transformation of benzylidene cyclobutanes to cyclopentanones.
18-20 In addition, they have been used for the synthesis of oxindoles from indoles
69 and for the desymmetrization of
meso-hydrobenzoins and the kinetic resolution of racemic hydrobenzoins.
70 They can also catalyze the stereoselective epoxidation of amphiphilic olefins; in the case of carboxylic acids, the carboxyl group can provide a directing effect as well as serve as a trigger for
in situ lactonization.
22
Appendix
Chemical Abstracts Nomenclature (Collective Index Number);
(Registry Number)
(14212-54-5)
1,3-dioxane (505-22-6)
(17540-04-4)
(18422-53-2)
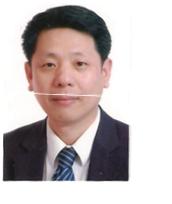 |
Yian Shi was born in Jiangsu, China in 1963.
He obtained his B.Sc.
degree from Nanjing University in 1983, M.Sc.
degree from University of Toronto with Professor Ian W.J.
Still in 1987, and Ph.D.
degree from Stanford University with Professor Barry M.
Trost in 1992.
After a postdoctoral study at Harvard Medical School with Professor Christopher Walsh, he joined Colorado State University as assistant professor in 1995, and he was promoted to associate professor in 2000 and professor in 2003.
His current research interests include the development of new synthetic methods, asymmetric catalysis, and synthesis of natural products.
|
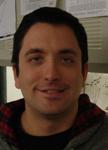 |
Thomas A.
Ramirez was born in Corpus Christi, Texas in 1980.
He received his B.S.
and M.S.
degrees from Texas A&M University-Kingsville in 2004 and 2006, respectively, working under the guidance of Professor Apurba Bhattacharya.
He is currently a graduate student in the research group of Professor Yian Shi at Colorado State University work on metal-catalyzed vicinal diamination/C-H amination methodologies.
Thomas is a Ronald E.
McNair Fellow and a BMS Minority Chemist Fellow (2009-2011).
|
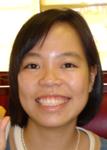 |
On Lo Andrea Wong was born in Hong Kong in 1981.
She moved to Honolulu, Hawai'i in 1994 and received her B.Sc.
degree in chemistry from the University of Hawai'i at Manoa in 2003.
She completed her doctoral dissertation in 2009 with Professor Yian Shi at Colorado State University; her research was focused on carbohydrate-derived asymmetric epoxidation.
She is currently a postdoctoral fellow in the laboratory of Professor Christopher Ackerson at Colorado State University working on the synthesis of monodispersed thiolate-protected gold nanoparticles.
|
Copyright © 1921-, Organic Syntheses, Inc. All Rights Reserved