Org. Synth. 2012, 89, 460-470
DOI: 10.15227/orgsyn.089.0460
Preparation of t-Butyl-3-Bromo-5-Formylbenzoate Through Selective Metal-Halogen Exchange Reactions
Submitted by Juan D.
Arredondo, Hongmei Li, and Jaume Balsells
1.
Checked by Julius R.
Reyes and Viresh H.
Rawal.
1. Procedure
t-Butyl-3,5-dibromobenzoate
(1).
An oven-dried 500-mL 3-necked round-bottomed flask, an oven-dried Teflon-coated oval-shaped magnetic stir bar (3.8 cm × 1.6 cm), and an oven-dried 125-mL pressure-equalizing addition funnel (fitted in the leftmost neck, capped with a rubber septum) are assembled hot and fitted with rubber septa on the center and right necks.
The apparatus is placed under high vacuum (< 1 mmHg) and allowed to cool to room temperature and subsequently maintained under a positive pressure of N2 using needle inlet through the septum on the rightmost neck.
Upon cooling, a thermometer is attached to the center neck using a Teflon adapter, and the apparatus is again evacuated under high vacuum.
It is then placed under a positive pressure of N2 and charged with 40.0 mL of toluene (Note 1), then cooled in a -15 °C acetone bath (Note 2).
A solution of n-butylmagnesium chloride (16.8 mL, 33.6 mmol, 0.42 equiv., 2.0M in THF) is added (Note 3) and the resulting grey solution is cooled to an internal temperature of -10 °C (Note 2), at which point 28 mL (67.5 mmol, 0.85 equiv, 2.41 M in hexanes) of n-butyllithium (Note 3) is added via a disposable syringe at such a rate so as to keep the internal temperature below -5 °C (approximately 30 min addition time) (Note 4).
The resulting milky white slurry is stirred at -10 °C for 30 min (Note 5).
During this time the addition funnel is charged with a solution of 25.00 g (79.4 mmol, 1.0 equiv) of 1,3,5-tribromobenzene in roughly 130 mL of toluene (Notes (Note 6) and (Note 7)).
Dropwise addition is initiated at such a rate that the internal temperature remains below -5 °C (approximately 45 min addition time).
The resulting dark maroon slurry is stirred at -10 °C for 1 h.
A solution of 22.7 g (104.0 mmol, 1.3 equiv) of di-tert-butyl dicarbonate (Note 8) in 30.0 mL of toluene is added slowly via cannula, keeping the internal temperature below -5 °C (approximately 40 min addition time).
After addition is completed, the reaction mixture is stirred at -10 °C for 2 h (Note 5).
At this point all septa are removed to facilitate ventilation of subsequently generated CO2, and the reaction is quenched by addition of 120 mL aqueous solution of 1M citric acid, which causes the internal temperature to rise from -14 °C to 6 °C (Note 9).
Upon warming to room temperature, the biphasic mixture is transferred to a 1-L separatory funnel and the organic phase is separated, then washed with an additional 120 mL of 1M aqueous citric acid.
The organic phase is dried over MgSO4 (40 g), filtered through a fritted glass funnel, and concentrated by rotary evaporation (40 °C, 20 mmHg).
The residue is further dried under high vacuum to afford a reddish-brown oil that is purified via flash column chromatography on silica gel (675 g, 9-cm diameter column, height of silica gel is 9 inches) to yield 2 as an orange solid (18.99 g, 71%) (Notes (Note 10), (Note 11), and (Note 12)).
t-Butyl-3-bromo-5-formylbenzoate
(2).
A 500-mL 3-necked round-bottomed flask with a Teflon-coated oval-shaped magnetic stir bar (3.8 cm × 1.6 cm) is flame-dried and charged with 18.91 g (56.3 mmol, 1.0 equiv) of purified dibromobenzoate 1 and 135 mL THF (Note 1).
The center neck is equipped with a thermometer using a Teflon adapter, and the left and right necks are fitted with rubber septa.
The resulting bright orange solution is cooled to an internal temperature of 0 °C in an ice-brine bath, and treated with 44 mL (96.8 mmol, 1.7 equiv, 2.2 M in THF) of iso-propylmagnesium chloride (Note 13) via disposable syringe at such a rate to keep the internal temperature below 21 °C (Note 14).
Upon complete addition, the solution is allowed to stir at room temperature for 1 h (Note 15).
The now blood-red solution is cooled back to 0 °C, and 11.0 mL (142.7 mmol, 2.5 equiv) of N,N-dimethylformamide (Note 13) is added via disposable syringe at such a rate to keep the internal temperature below 25 °C (Note 16).
The deep red reaction mixture is then stirred at room temperature for 30 min, then diluted with 100 mL of tert-butyl methyl ether (Note 13) and quenched by addition of 100 mL of saturated aqueous NH4Cl (Note 17).
The two layers are separated in a 1-L separatory funnel, and the organic phase is washed with an additional 100 mL of saturated aqueous NH4Cl.
The aqueous layers are then back-extracted once with 100 mL of ethyl acetate.
The combined organic layers are dried over MgSO4 (40 g), filtered through a fritted glass funnel, and concentrated by rotary evaporation (25 °C, 20 mmHg) to afford crude 2, a bright orange/brown oil.
Purification by flash column chromatography on silica gel (675 g, 9-cm diameter column, height of silica gel is 9 inches) affords 2 as a thick golden yellow oil that crystallizes upon standing (12.96 g, 81% yield) (Notes (Note 18) and (Note 19)).
2. Notes
1.
Toluene and tetrahydrofuran (both of Optima® grade) were purchased from Fisher Scientific and dried by passing through an activated alumina column solvent purification system (Innovative Technology Inc.
Pure Solv
TM).
2.
The bath temperature is maintained using a Neslab CC-65 immersion cooler.
3.
n-Butylmagnesium chloride (2.0M in THF) and
n-butyllithium (2.5M in hexanes) were purchased from Sigma-Aldrich and titrated prior to use.
n-Butyl magnesium chloride arrived as a cloudy, grey solution, and titrated to be 2.0M;
n-butyllithium arrived as a cloudy, yellow solution, and titrated to be 2.41M.
4.
The reaction mixture changes from grey to a milky white slurry.
5.
The checkers followed reaction progress by quenching an aliquot with saturated aqueous NH
4Cl and taking its
1H NMR spectrum.
The submitters monitored reaction progress by quenching an aliquot with methanol and determined progress by HPLC analysis on an Ascentis Express C18 column (10 cm × 4.6 cm; flow rate 1.8 mL/min; mobile phase acetonitrile/ 0.1% aqueous H
3PO
4, 10% to 95% acetonitrile over 6 min and hold 95% for 2 min; UV detection at 254 nm), diagnostic peaks t
R (min) at 4.6 (toluene), 5.6 (dibromobenzene), 6.4 (tribromobenzene), 6.9 (
t-butyl-dibromobenzoate).
6.
1,3,5-Tribromobenzene (98%) was purchased from Sigma-Aldrich and used as received.
7.
IMPORTANT: 1,3,5-Tribromobenzene is sparingly soluble in toluene at room temperature.
Thus, prior to generation of the ate complex, the checkers dissolved the tribromobenzene in toluene by heating to 90 °C and cooling the solution back to room temperature.
Once dissolved, the tribromobenzene remains in solution for some time.
Upon dissolution of 1,3,5-tribromobenzene in toluene, some dark solids remained.
The solids were removed by filtration for the first run (half-scale), but not for the second run (full-scale).
The presence of these solids had no effect on the success of the reaction.
8.
Di-
tert-butyl dicarbonate (reagent grade, 97%) was purchased from Sigma-Aldrich and used as received.
9.
Citric acid monohydrate (reagent grade) was purchased from Fisher Scientific.
10.
The checkers also obtained the crude product in quantitative yield, but despite numerous attempts the crude sample gave low yields when used directly in the next step.
The submitters reported a quantitative yield of the crude product that was deemed to be sufficiently pure (by HPLC, see Note 5 for method conditions) for use in the next step.
The submitters purified the crude material by column chromatography using 95:5 hexanes:ethyl acetate as the eluent and obtained the purified product in 97% yield.
11.
The checkers purchased silica gel from SiliCycle (SiliaFlash® P60 40-63μm). The column was wet-packed and eluted with 6:1 hexanes:dichloromethane. One large fraction (1 L) and thirty-six smaller fractions (125-150 mL) were collected. The product was found (by TLC) in fractions 9-28, which were combined and concentrated by rotary evaporation (25 °C, 20 mmHg) and then under high vacuum (< 1 mmHg) to yield
1, with the following physical properties: mp: 58-61 °C; R
f = 0.32 (5:1 hexanes:dichloromethane, 254 nm UV lamp);
1H NMR
pdf(500 MHz, CDCl
3) δ (ppm): 1.59 (s, 9 H), 7.81 (t,
J = 2 Hz, 1 H), 8.03 (d,
J = 2 Hz, 2 H);
13C NMR
pdf(125 MHz, CDCl
3) δ (ppm): 28.1, 82.5, 122.8, 131.2, 135.2, 137.7, 163.0; IR (neat film, NaCl): 742.3, 764.7, 847.8, 1135.4, 1165.2, 1282.0, 1368.7, 1451.1, 1558.4, 1719.2, 2978.0 cm
−1.
HRMS
m/z calcd.
for (C
11H
12O
2Br
2)Na
+: 356.9096; found (electrospray)
m/z: Not detected.
CHN analysis for C
11H
12O
2Br
2: C, 39.32; H, 3.60.
Found (duplicate): C, 39.45/39.51; H, 3.65/3.74.
12.
On half scale, the checkers obtained
1 in 74% yield.
13.
iso-Propylmagnesium chloride (2.0M in THF),
N,N-dimethylformamide (anhydrous, 99.8%) and
tert-butyl methyl ether (reagent grade, 98%) were purchased from Sigma-Aldrich.
iso-Propylmagnesium chloride was titrated prior to use and found to be 2.2M.
N,N-dimethylformamide and
tert-butyl methyl ether were used as received.
14.
The checkers found the internal temperature to rise to ~10 °C, accompanied by darkening of the reaction mixture to black/orange.
The submitters noted the reaction temperature to rise to 18 °C, with darkening of the reaction mixture.
15.
Progress of the reaction was monitored as described in Note 5.
The submitters monitored complete consumption of starting material by quenching an aliquot of the reaction mixture with methanol and determined progress by HPLC analysis on an Ascentis Express C18 column (10 cm × 4.6 cm; flow rate 1.8 mL/min; mobile phase acetonitrile/ 0.1% aqueous H
3PO
4, 10% to 95% acetonitrile over 6 min and hold 95% for 2 min; UV detection at 254 nm), diagnostic peaks t
R (min) at 6.1 (
t-butyl-bromobenzoate), 5.7 (aldehyde), 6.9 (
t-butyl-dibromobenzoate).
16.
A moderate exotherm is observed, with the internal temperature rising to 20-22 °C.
17.
The submitters quenched the reaction with a 1M citric acid solution.
18.
The sample was loaded on a wet-packed column and eluted with 9:1 hexanes:diethyl ether.
One large fraction (1 L) and forty smaller fractions (100 mL) were collected.
The product was found (by TLC) in fractions 16-35, which were combined and concentrated by rotary evaporation (25 °C, 20 mmHg) and then under high vacuum (< 1 mmHg) to yield
2: mp: 61-63 °C; R
f = 0.28 (9:1 hexanes:diethyl ether, 254 nm UV lamp);
1H NMR
pdf(500 MHz, CDCl
3) δ (ppm): 1.63 (s, 9 H), 8.16 (d,
J = 2 Hz, 1 H), 8.34 (d,
J = 2 Hz, 1 H), 8.38 (d,
J = 2 Hz, 1 H), 10.02 (s, 1 H);
13C NMR
pdf(125 MHz, CDCl
3) δ (ppm): 28.0, 82.6, 123.2, 129.6, 134.8, 135.0, 137.7, 137.7, 163.1, 189.9; IR (neat film, NaCl): 664.9, 719.4, 766.1, 845.2, 889.4, 1155.1, 1200.8, 1246.6, 1296.5, 1369.1, 1456.6, 1575.1, 1706.4, 2978.4 cm
−1.
HRMS
m/z calcd.
for (C
12H
13O
3Br)Na
+: 306.9940; found (electrospray)
m/z: 306.9940.
CHN analysis for C
12H
13O
3Br: C, 50.55; H, 4.60.
Found (duplicate): C, 50.67/50.38; H, 4.71/4.68.
19.
On half-scale, the checkers obtained an 84% yield of
2.
The submitters reported obtaining
2 in 91% yield.
Handling and Disposal of Hazardous Chemicals
The procedures in this article are intended for use only by persons with prior training in experimental organic chemistry. All hazardous materials should be handled using the standard procedures for work with chemicals described in references such as "Prudent Practices in the Laboratory" (The National Academies Press, Washington, D.C., 2011 www.nap.edu). All chemical waste should be disposed of in accordance with local regulations. For general guidelines for the management of chemical waste, see Chapter 8 of Prudent Practices.
These procedures must be conducted at one's own risk. Organic Syntheses, Inc., its Editors, and its Board of Directors do not warrant or guarantee the safety of individuals using these procedures and hereby disclaim any liability for any injuries or damages claimed to have resulted from or related in any way to the procedures herein.
3. Discussion
Transition metal-catalyzed carbonylation reactions have become the method of choice to prepare benzoate esters from aryl bromides.
2 These methods represent an improvement over other methodologies in terms of yields, reaction conditions and substrate scope.
Alternative methods involving metallation or metal-halogen exchange, followed by trapping the resulting organometallic species with carbon dioxide, methyl chloroformate
3 or dimethyl carbonate,
4 often require cryogenic conditions and have limited compatibility to functional groups.
One of the limitations of transition metal-catalyzed carbonylations is the lack of selectivity for the monocarbonylation of substrates containing multiple halogen substituents.
On these substrates, the product of the first carbonylation reaction is more reactive to carbon-halogen bond insertion than the starting aryl halide and it becomes very difficult to achieve selectivity in the reaction.
5
Researchers have looked for solutions to this selectivity problem.
Cassar and coworkers
6 showed that, under very controlled Pd-catalyzed carbonylation conditions, it was possible to prepare 4-bromobenzoic acid in 90% yield from 1,4-dibromobenzene.
The key to obtaining high selectivity in this reaction was a slow addition of the substrate under phase transfer conditions.
Under these reaction conditions, the product of the first carbonylation reaction was extracted as a carboxylate salt into the aqueous layer, thus being unavailable for a second palladium insertion.
Lee and coworkers
7 attempted to improve the selectivity using a metallation approach.
They carried out the direct metallation of 1,4-dibromobenzene with 2.5 equivalents of magnesium under sonication conditions, followed by trapping of the resulting Grignard with diethyl dicarbonate in the presence of Lewis acids.
With this method, they obtained ethyl 4-bromobenzoate in 92% yield, with only 3% of diethyl terephthalate.
Lower selectivities were obtained with other dibromides studied.
In an effort to improve the performance and selectivity of this transformation, we turned our attention to a report from Iida, Mase and coworkers,
8 who showed good reactivity and very high selectivities for metal-halogen exchange reactions of dibromoarenes using lithium tri-
n-butylmagnesium ate complex.
We later discovered that di-
t-butyl dicarbonate is an excellent electrophile to trap triarylmagnesium ate complexes affording the desired
t-butyl esters with complete selectivity and in very high yields.
Although chemists tend to rely mostly on methyl or ethyl esters, the use of t-butyl esters may have advantages in some cases.
The possibility of t-butyl ester hydrolysis under acidic conditions makes these compounds complimentary to simpler esters such as methyl or ethyl.
Within the context of this chemistry, we have successfully used the t-butyl moiety as a protecting group for the ester functionality in subsequent Grignard chemistry.
This is exemplified in the procedure above by the reaction of t-butyl-3,5-dibromobenzoate obtained by our method with i-propylmagnesium chloride followed by addition to dimethylformamide.
This methodology has been extended to other di and tri-haloarenes and heteroarenes as shown in the table below.
9 Very high selectivities for the metal-halogen exchange reaction were observed in all the substrates examined and yields for the desired ester products were uniformly high except for the pyridine substrates.
Table 1. n-Bu3MgLi-mediated mono-carboxylation of di and tribromoarenes and heteroarenes.
Appendix
Chemical Abstracts Nomenclature (Collective Index Number);
(Registry Number)
t-Butyl-3,5-dibromobenzoate; (5342-97-2)
n-Butylmagnesium chloride; (693-04-9)
n-Butyllithium; (109-72-8)
Di-tert-butyl dicarbonate; (24424-99-5)
1,3,5-Tribromobenzene; (626-39-1)
t-Butyl-3-bromo-5-formylbenzoate; (1018948-99-6)
iso-Propylmagnesium chloride; (1068-55-9)
N,N-Dimethylformamide; (
68-12-2)
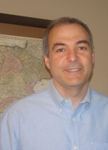 |
Jaume Balsells received his PhD from the University of Barcelona in 1998 under the supervision of Professor Miquel A.
Pericas.
After completing postdoctoral work with Professors Patrick J.
Walsh and Gary A.
Molander at the University of Pennsylvania, he joined the Process Research Department at Merck & Co., where he is currently an Associate Director.
His Research interests include the development of practical synthetic strategies and methodology for the preparation of pharmaceutical intermediates, Heterocyclic Chemistry and Green Chemistry.
|
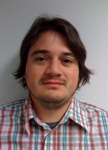 |
Juan D.
Arredondo was born in Englewood, New Jersey.
He received his undergraduate Chemistry degree in 2001 from Rutgers University under the guidance of Professor Kathryn Uhrich were he worked on the synthesis of derived salicylate-based poly(anhydride esters).
He obtained his M.S.
in Chemistry in 2006 from the University of Pittsburgh with Professor Peter Wipf.
His research focused on zirconium-catalyzed asymmetric carboalumination.
That same year he joined the Process Research Department of Merck & Co.
|
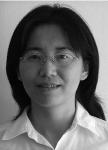 |
Hongmei Li received her B.Sc.
in chemistry at the Dalian University of Technology in 1999 and her M.Sc.
degree in inorganic chemistry (2002) at the Peking University with Professor Jianhua Lin.
She began her Ph.D.
at the University of Pennsylvania under the direction of Professor Patrick J.
Walsh in 2002, during which she worked on the catalytic asymmetric addition of organozinc reagents to ketones and application of bimetallic intermediates in organic synthesis.
In 2007, she joined the Department of Process Research at Merck & Co.
Inc., Rahway, NJ.
Her research interests focus on design and development of practical synthetic methodology and processes for the synthesis of pharmaceutical intermediates and drug candidates.
|
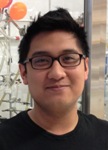 |
Julius R.
Reyes received his B.S.
in Chemistry in 2010 from the University of California, Berkeley, where he worked with Professors Richmond Sarpong and Andrew Streitwieser.
The following summer he joined the labs of Prof.
Viresh Rawal at the University of Chicago and is currently working on the development of novel hydrogen bond donor catalysts.
|
Copyright © 1921-, Organic Syntheses, Inc. All Rights Reserved