Org. Synth. 2012, 89, 491-500
DOI: 10.15227/orgsyn.089.0491
Preparation of Cyclobutenone
Submitted by Audrey G.
Ross, Xiaohua Li, and Samuel J.
Danishefsky
1.
Checked by Markus Roggen and Erick M.
Carreira
.
1. Procedure
Caution! This procedure should be carried out in an efficient fume hood.
Precautions should be taken to avoid inhalation of or contact with red mercury oxide
which is highly toxic
bromine
which is corrosive
toxic
tri-n-butylamine
which is toxic especially to aquatic organisms
cyclobutenone
which readily polymerizes
has unstudied health effects.
3-Bromocyclobutanone.
A 500-mL, single-necked, round-bottomed flask with a 24/40 ground glass joint is equipped with a 6-cm Teflon-coated magnetic stir bar (Note 1) and charged with 3-oxocyclobutanecarboxylic acid (20 g, 175 mmol, 1 equiv) (Note 2).
Dichloromethane is added (250 mL, 0.7 M) (Note 3) to dissolve the acid at ambient temperature (23 °C).
While stirring gently at 150 rpm, anhydrous magnesium sulfate (21.1 g, 175 mmol, 1 equiv) is added to the reaction, followed carefully by red mercury oxide (56.9 g, 263 mmol, 1.5 equiv).
A reflux condenser is assembled (Note 4) and capped with a 24/40 rubber septum, through which the reaction is maintained under argon atmosphere (Note 5).
The heterogeneous mixture is then stirred at 250-300 rpm and brought to vigorous reflux (oil bath temperature 46 °C) for 5 to 10 min to ensure freely flowing solids (Note 6).
Separately, neat bromine (13.5 mL, 263 mmol, 1.5 equiv) is taken up in a glass-syringe with a needle approximately as long as the reflux condenser (12 inches).
The needle is threaded through the rubber septum at the top of the reflux condenser such that bromine will be released near or at the bottom of the condenser (Note 7).
Bromine is carefully added dropwise over about 30 min to control the release of CO2 (g).
(Figure 1) Three disposable 18-gauge needles are inserted into the septum to vent the reaction for the duration of bromine addition, and can be adjusted as needed.
Figure 1. Reaction Set-up for Step A
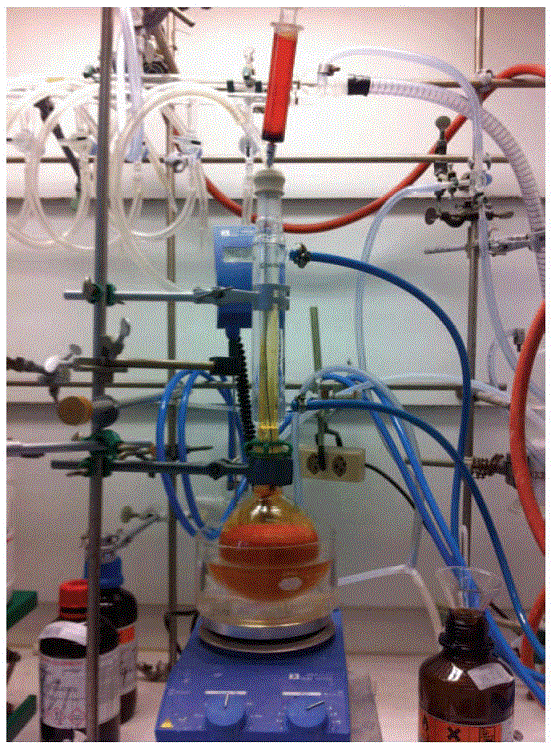
The reaction color gradually fades from bright orange to pale orange-yellow.
After 3 h the reaction progress is checked by TLC (Note 8).
As TLC indicates incomplete conversion, additional bromine (7 mL, 136 mmol, 0.775 equiv) is added via syringe and the reaction is continued for a total of 6 h.
After completion the reaction is cooled to ambient temperature (23 °C) and filtered through a pad of celite and silica gel (Note 9) with the aid of additional methylene chloride (250 mL).
The clear, colorless filtrate is placed in a 1-L separatory funnel and washed with saturated aqueous sodium bicarbonate (3 × 200 mL).
The combined milky aqueous washes are back-extracted with methylene chloride (200 mL) (Note 10).
The combined organic extracts are washed with water (200 mL) and then brine (200 mL).
The solution is dried over approximately 5 g of magnesium sulfate, filtered, and concentrated by rotary evaporator (25 °C, 100 to 30 mmHg) to give crude bromide 1 as a clear pale yellow liquid (23.6 g, 90% yield) (Notes (Note 11), (Note 12), and (Note 13)).
Cyclobutenone.
A 250-mL, single-necked, round-bottomed flask equipped with a 5-cm Teflon-coated magnetic stir bar (Note 1) is charged with tri-n-butylamine (54.5 mL, 228 mmol, 2 equiv) (Notes (Note 2) and (Note 14)) at ambient temperature (23 °C).
Neat 3-bromocyclobutenone 1 is added to the amine dropwise over 30 min (17.0 g, 114 mmol, 1 equiv) and settles into a lower layer.
The reaction mixture is stirred for 1 h at ambient temperature, and can be stirred for 2 h without adverse effect.
No precautions are taken to exclude light or monitor reaction progress by TLC.
A 100-mL, single-necked, pear-bottomed flask is connected to the reaction flask via a short path distillation head with Vigreux indents (Notes (Note 1) and (Note 15)).
This receiving flask is cooled to -78 °C in a dry ice/acetone bath, and the reaction flask is placed in an oil bath held constant at 25 °C.
(Figure 2) As the reaction flask is vigorously stirred at 250 rpm, pressure is carefully reduced to 2.5 mmHg and held for 20 min to ensure that cyclobutenone 2 does not foam over too rapidly (Note 16).
At this time the receiving flask contains semi-frozen cyclobutenone, which rapidly melts to a clear and faint yellow liquid upon moving the receiving flask to ambient temperature (4.5-4.7 g, 58-60% yield) (Note 17).
In this preparation, crude melted cyclobutenone 2 is dissolved in deuterated chloroform for use as a 1M solution (Notes (Note 18) and (Note 19)).
If desired, the cyclobutenone 2 can be briefly stored neat at -78 °C, while the product purity is quickly checked by 1H NMR (Notes (Note 20), (Note 21), and (Note 22)).
Figure 2. Reaction set-up for Step B
2. Notes
1.
Glassware and stir bars were dried for at least 12 h in a 140 °C oven, cooled to ambient temperature under reduced pressure, then backfilled with argon atmosphere.
2.
3-Oxocyclobutanecarboxylic acid was purchased from Advanced ChemBlocks, Inc.
and used without further purification.
As a precaution the acid was stored in a refrigerator at 0 °C.
Alternatively, the acid can be prepared according to literature procedure.
2 All other chemicals were used as received and stored at ambient temperature on the benchtop.
Anhydrous magnesium sulfate and deuterated chloroform were purchased from Fisher Scientific International, Inc.
SiliCycle SiliaFlash P60 (230-400 mesh) was used.
All other chemicals were purchased from Aldrich Chemical Company, Inc.
3.
Methylene chloride (99.5%, stabilized, certified ACS) was purchased from Fisher Scientific International, Inc., and freshly distilled over calcium hydride before use.
For ease of scale, this modified Hunsdiecker reaction is notably more concentrated than those previously reported.
3 Although some conversion of methylene chloride to bromodichloromethane (
1H NMR δ 7.19 in CDCl
3) occurs under the reaction conditions, methylene chloride is used in preference to carbon tetrachloride for reduced toxicity and easier isolation of the volatile bromide
1.
4.
The reflux condenser was dried for at least 12 h in a 140 °C oven, then cooled to ambient temperature.
The condenser was attached to the reaction flask via a 24/40 ground glass joint lubricated with high-vacuum grease purchased from Dow Corning.
5.
The submitters recommend using a wide-gauge needle to provide pressure exchange via the argon inlet.
6.
Vigorous stirring and a large stir bar are crucial for this reaction.
One can break up clumps of solids with a large spatula if needed, ideally before adding bromine.
7.
Excess reagent is required to account for volatility of bromine, and that some bromine and red mercury oxide will react with the solvent.
8.
Reaction progress can be monitored by TLC (bromide
1 R
f = 0.63 in CH
2Cl
2, visualized with KMnO
4 stain; 3-oxocyclobutanecarboxylic acid R
f = baseline in CH
2Cl
2, R
f = 0.41 in diethyl ether, visualized with KMnO
4 stain).
To reduce exposure risk, the submitters advise that the reaction as allowed to proceed for a set time of 3 h.
The submitters report full conversion after 3 h A reaction allowed to run for 1.5 h provided bromide
1 in a yield of 54%.
9.
Vacuum filtration performed on a large (>150 mL) fritted glass funnel filled with a 1-cm layer of celite and silica.
Substantial solids are collected and treated as mercury-containing hazardous waste.
10.
Spent aqueous washes are combined with and treated as mercury-containing hazardous waste.
11.
Crude bromide
1 can be carried forward, since non-volatile impurities will not contaminate cyclobutenone
2.
For characterization purposes, a sample of the volatile bromide
1 was quickly purified by silica gel chromatography to remove trace bromodichloromethane and an impurity at R
f = 0.44 (CH
2Cl
2, visualized with KMnO
4 stain).
Bromide
1 (0.2 g) was charged on a small glass column (1 × 10 cm) containing 1.23 g silica gel (see Note 2).
Product was eluted with 10 mL of 9:1 pentane/diethyl ether, and collected in 0.6 mL fractions.
Product eluted in fractions 8 - 11.
Distillation of bromide
1 is reported in the literature (22-25 °C at 0.5-0.25 mmHg).
4
12.
3-Bromocyclobutanone (
1) characterization (purified material): R
f = 0.63 (CH
2Cl
2, visualized with KMnO
4 stain);
1H-NMR
pdf(400 MHz, CDCl
3) δ: 3.43-3.52 (m, 2 H), 3.72-3.81 (m, 2 H), 4.54 (tt,
J = 7.9, 5.0 Hz, 1 H).
13C NMR
pdf(101 MHz, CDCl
3) δ: 29.0, 60.4, 203.2; IR (neat): 1787, 1372, 1233, 1139, 1084, 996, 967, 838, 700 cm
−1.
MS
m/
z (relative intensity): 41.0339 (100%), 69.0335 (60.99%), 118.9489 (5.83%), 120.9484 (6.73%).
Anal.
Calcd for C
4H
5BrO: C, 32.25; H, 3.38.
Found: C, 32.07; H, 3.42 (The checkers found it necessary to keep purified
1 at -78 °C prior to elemental analysis.)
13.
Bromide
1 is moderately stable to neat storage in a 0 °C refrigerator.
Although the material develops a yellow to orange color, NMR analysis reveals useable stability for up to two weeks.
When left exposed to light on the benchtop at ambient temperatures, neat samples of bromide
1 decomposed to a black tar within two days.
14.
Excess amine (2 equiv) is used in this procedure as solvent and reagent.
Diethylene glycol diethyl ether (diethyl carbitol) can be used as a solvent to allow stoichiometric amine and a homogeneous solution.
3,4 To obtain more pure final product, the ether is forgone as suggested in an earlier preparation of cyclobutenone.
4
15.
The short-path distillation head was dried for at least 12 h in a 140 °C oven, then cooled to ambient temperature.
All ground glass joints were lubricated with high-vacuum grease purchased from Dow Corning.
16.
The boiling point of tri-
n-butylamine is 216 °C.
The boiling point range of diethylene glycol diethyl ether (diethyl carbitol) is 180-190 °C.
If one prefers to use the ether in order to use stoichiometric amine, more care or a second distillation may be necessary to obtain optimally pure final product.
17.
Cyclobutenone
2 characterization: R
f = 0.43 (4:1 pentane/ether, diffuse spot visualized with KMnO
4 stain);
1H-NMR
pdf(400 MHz,) δ: 3.34 (t,
J = 0.8 Hz, 2 H), 6.15 (dt,
J = 2.8, 0.7 Hz, 1 H), 8.21 (dt,
J = 2.9, 0.9 Hz, 1 H);
13C NMR
pdf(101 MHz; CDCl
3) δ: 51.1, 141.4, 162.6, 189.9; [M] calcd for C
4H
4O: 68.0262.
Found: 68.0252.
IR (in CDCl
3): 2931, 1770, 1524, 1283, 1037 cm
−1.
GC analysis of a 1M solution of cyclobutenone in CDCl
3 was performed on a Shimadzu GC 17A ver.
3 instrument using a SupelCo SPB-5 column (30.0 m × 0.25 mm) with a flame ionization detector.
The assay is held steady at 50 °C after injection at 220 °C.
The observed retention times are 3.99 min for cyclobutenone, and 5.88 min for deuterated chloroform.
18.
Solutions of cyclobutenone in deuterated chloroform are quite stable to storage, with no appreciable decomposition noted after one month in a 0 °C refrigerator.
Substantial decomposition is noted by eight months under the same storage conditions.
Solutions were stored in deuterated chloroform bottles and did not require argon atmosphere or seals.
Other solvents, concentrations, and temperatures for storage were not examined.
19.
All waste from this reaction is treated as hazardous amine waste toxic to aquatic organisms, including washes of distillation glassware.
20.
Neat cyclobutenone
2 rapidly polymerizes at ambient temperature, and will polymerize at -78 °C.
The submitters have kept cyclobutenone
2 neat at -78 °C for about 20 min without appreciable unwanted reaction.
Cyclobutenone
2 smells like superglue and has irritating vapors.
21.
If necessary, a second distillation can be performed to leave behind less volatile contaminants such as tri-
n-butylamine.
This must be done rapidly to minimize polymerization and loss of yield.
22.
The submitters report a separate run which used 23.0 g of bromide
1 provided cyclobutenone
2 (6.86 g, 100.7 mmol, 65% yield) under the same reaction conditions.
Handling and Disposal of Hazardous Chemicals
The procedures in this article are intended for use only by persons with prior training in experimental organic chemistry. All hazardous materials should be handled using the standard procedures for work with chemicals described in references such as "Prudent Practices in the Laboratory" (The National Academies Press, Washington, D.C., 2011 www.nap.edu). All chemical waste should be disposed of in accordance with local regulations. For general guidelines for the management of chemical waste, see Chapter 8 of Prudent Practices.
These procedures must be conducted at one's own risk. Organic Syntheses, Inc., its Editors, and its Board of Directors do not warrant or guarantee the safety of individuals using these procedures and hereby disclaim any liability for any injuries or damages claimed to have resulted from or related in any way to the procedures herein.
3. Discussion
The difficulty in preparing and handling cyclobutenone has precluded its usage for many years.
In 1954, Cope and coworkers suggested its generation as a byproduct from the decomposition of 2,4,6-cyclooctatrien-1-one Diels-Alder adducts, noting a volatile carbonyl-containing compound with a sharp odor.
5 Soon after, Vogel and Hasse attempted to liberate the proposed cyclobutenone by pyrolysis of the methyl ketal of the same adduct.
6 Treatment of isolated cyclobutenone dimethyl ketal with aqueous acid gave a mixture of 3-hydroxycyclobutanone, 3-methoxycyclobutanone, and 1,3,5-triactylbenzene.
Thus isolation and direct confirmation of cyclobutenone was thwarted by its reactivity.
It was not until 1971 that cyclobutenone was first conclusively prepared, when Sieja was able to access the parent compound through two independent routes based on allene and ketene.
4 Despite its curious structure and potential applications due to its inherent strain, cyclobutenone received little attention in the following decades.
It is feasible that the challenging synthetic routes and inability to prevent polymerization during overnight storage at -78 °C ensured that cyclobutenone would remain of limited practical utility.
In 2004, Helal and coworkers heeded Sieja's suggestion to prepare cyclobutenone for immediate use.
7They treated 3-acetoxycyclobutanone with 1,8-diazabicyclo[5.4.0.]-undec-7-ene (DBU) and 4-nitroimidazole, resulting in a net exchange of acetate with 4-nitroimidazole.
Given the generally weak reactivity of 4-nitroimidazole in displacement reactions as noted by the authors, it is presumed that this transformation occurs by the
in-situ generation of cyclobutenone.
To make cyclobutenone available for basic study and uses in which it cannot be conveniently generated
in-situ, Danishefsky and Li developed an updated synthesis of cyclobutenone in 2010.
3 This procedure represents an optimized and moderately scaled-up version of said route, by which cyclobutenone can be prepared in two steps from commercial material.
The first step is a mild variant of a Cristol-Firth modified Hunsdiecker reaction.
8,9 Cyclobutenone
2 is then prepared by elimination of the newly formed bromide
1, at which time the product is distilled out for isolation.
Importantly, it was found that cyclobutenone can be kept as a stock solution in deuterated chloroform, enabling significantly longer storage, further characterization, simple measurement and handling of the elusive title compound.
Thus cyclobutenone can be prepared in high purity in approximately 58% yield.
The ability to prepare, isolate, and store cyclobutenone has allowed its study as a highly reactive dienophile in Diels-Alder additions,
a subject of experimental and computational interest.
3,10
Appendix
Chemical Abstracts Nomenclature (Collective Index Number);
(Registry Number)
3-Oxocyclobutanecarboxylic acid ((23761-23-1)
Mercury (II) oxide red (21908-53-2)
Bromine (7726-95-6)
3-Bromocyclobutanone (23761-24-2)
Tri-n-butylamine (102-82-9)
Cyclobutenone; 2-cyclobuten-1-one (32264-87-2)
Deuterated chloroform; chloroform-d; deuterochloroform (8
65-49-6)
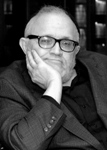 |
Samuel J.
Danishefsky received his B.S.
degree at Yeshiva University.
He did graduate research under the late Professor Peter Yates, and then joined the laboratory of Professor Gilbert Stork at Columbia University as an NIH Postdoctoral Associate.
His first academic position was at the University of Pittsburgh.
In 1980, he moved to Yale University and became Sterling Professor in 1990.
In 1993, Professor Danishefsky moved to New York as Professor of Chemistry at Columbia University and Eugene Kettering Chair and Head of the Laboratory of Bioorganic Chemistry at Memorial Sloan-Kettering Cancer Center.
He shared the Wolf Prize in Chemistry with Professor Stork in 1996. |
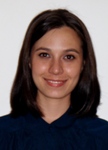 |
Audrey G.
Ross received her B.S.
in chemistry from the University of California, Los Angeles in 2007, where she did undergraduate research under the supervision of Professor Kendall N.
Houk.
She is currently pursuing her Ph.D.
at Columbia University in the laboratory of Professor Samuel Danishefsky.
In her graduate work, she has studied the total synthesis of natural products, and cyclobutenone in Diels-Alder and intramolecular Diels-Alder reactions.
Audrey is a National Science Foundation Predoctoral Fellow. |
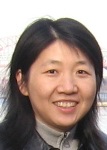 |
Xiaohua Li received her B.S.
in chemistry from Tianjin University in 1998 and her M.S.
in Chemical Engineering from Beijing Institute of Technology in 2001, China.
She came to the United States afterwards and pursued her Ph.D.
in organic chemistry under the supervision of Professor David Mootoo at Hunter College, CUNY.
Her research focused on the development and application of new spiroketalization methods in natural product synthesis.
Later she worked with Professor Samuel Danishefsky at Columbia University as a postdoctoral fellow on the preparation and use of cyclobutenone as dienophile in Diels-Alder reactions.
Currently she is a visiting assistant professor at University of Toledo. |
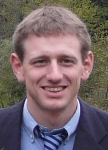 |
Marcus Roggen received his M.
Sci.
in Chemistry with First Class Honors in 2008 from Imperial College in London.
During his undergraduate studies he performed research at the Nanyang Technological University in Singapore under the direction of Roderick Bates and at the ETH in Zurich University under the direction of Prof.
Erick Carreira.
In 2008 he entered the Ph.D.
program at the ETH. |
Copyright © 1921-, Organic Syntheses, Inc. All Rights Reserved