Org. Synth. 2013, 90, 41-51
DOI: 10.15227/orgsyn.090.0041
Preparation of (E)-N,N-Diethyl-2-styrylbenzamide by Rh-Catalyzed C-H Activation
Submitted by Frederic W. Patureau, Tatiana Besset, and Frank Glorius
1.
Checked by Takaaki Harada and Tohru Fukuyama.
1. Procedure
(E)-N,N-Diethyl-2-styrylbenzamide: A flame-dried, 200-mL, three-necked round-bottomed flask is equipped with 2.5 cm rod-shaped, Teflon-coated, magnetic stirbar, glass stopper, rubber septum and reflux condenser fitted with argon inlet (Note 1). The flask is flushed with argon and charged with [Cp*RhCl2]2 (45.8 mg, 0.074 mmol, 0.25 mol%) (Note 2), AgSbF6 (102 mg, 0.30 mmol, 1.00 mol%) (Note 3), Cu(OAc)2 (11.3 g, 62.2 mmol, 2.10 equiv) (Note 4) and 1,4-dioxane (30 mL) (Note 5). N,N-Diethylbenzamide (5.25 g, 29.6 mmol, 1 equiv) (Note 6) in 1,4-dioxane (10 mL) is added via cannula and styrene (5.11 mL, 44.4 mmol, 1.50 equiv) (Note 7) is added via syringe into the resulting blue-green suspension. The rubber septum is changed to a glass stopper and the stirred suspension is heated in an oil bath (125 °C) for 23 h (Note 8). The reaction mixture is allowed to cool to room temperature, gravity filtered through a cotton plug into a 500-mL round bottomed flask and rinsed with toluene (150 mL) (Note 9). The resulting green solution is concentrated by rotary evaporation (40 °C water bath, 40 mmHg,). Toluene (2 x 200 mL) is added and then evaporated twice (Note 10) (40 °C water bath, 40 mmHg). The crude is filtered through a short plug of silica gel (Notes 11 and 12), washed with EtOAc (500 mL), and concentrated by rotary evaporation (40 °C water bath, 70 mmHg) to provide a yellow viscous oil (Note 13). This material is purified by silica gel column chromatography (Notes 14 and 15). The combined eluent is concentrated by rotary evaporation (40 °C water bath, 70 mmHg) and then submitted to high vacuum (0.3 mmHg) at 80 °C for 24 h (Note 16) to furnish 7.62 g (27.3 mmol, 92%) of (E)-N,N-diethyl-2-styrylbenzamide as a light yellow viscous oil (Note 17).
2. Notes
1.
The submitters used a dry Schlenk-tube (capacity: 166 mL, diameter: 45 mm) with a screw cap equipped with a 2.5 cm magnetic stir bar under an argon atmosphere. The submitters have shown that air could serve as terminal oxidant; nevertheless, the reaction is less effective in moist conditions, presumably due to the competitive binding of water to the active metal coordination sites. Therefore, use of an argon atmosphere is recommended.
2.
The submitters purchased [Cp*RhCl
2]
2 from Heraeus and stored the catalyst in a glovebox under argon. The checkers purchased [Cp*RhCl
2]
2 from Aldrich and used the material as received.
3.
The submitters purchased AgSbF
6 from ABCR and stored the material in a glovebox under argon. The checkers purchased AgSbF
6 from Aldrich and used the material as received.
4.
The submitters prepared Cu(OAc)
2 from Cu(OAc)
2(H
2O), which was purchased from Aldrich, by heating at 100 °C under high vacuum (approx. 0.1 mmHg) for 48 h. The checkers prepared Cu(OAc)
2 from Cu(OAc)
2(H
2O), which was purchased from Aldrich, by heating at 100 °C under vacuum (2 mmHg) for 72 h.
5.
The checkers purchased 1,4-dioxane from Aldrich and the solvent was stored over activated 4Å molecular sieves.
6.
The submitters prepared
N,N-diethylbenzamide, R
f = 0.3 (pentane/ethyl acetate 8:2), a light yellow liquid, from a simple condensation of Et
2NH with benzoyl chloride. The checkers purchased
N,
N-diethylbenzamide (98.0+%) from Wako and used the material as received.
7.
The submitters purchased styrene from Acros and used as received. The checkers purchased styrene (>99.0%) from Tokyo Chemical Industry Co., Ltd. and used it as received.
8.
The consumption of the starting material was monitored by TLC analysis on Merck silica gel 60 F254 plates (0.25 mm, glass-backed, visualized with 254 nm UV lamp) using 20% acetone in toluene as an eluant.
N,
N-Diethylbenzamide (starting material) had R
f = 0.49 (UV active) and (
E)-
N,
N-Diethyl-2-styrylbenzamide had R
f = 0.58 (UV active)
9.
Large brown-red precipitate that is typical for Cu
I/Cu
0 species is removed.
10.
Azeotropic removal of styrene and 1,4-dioxane was performed in order to simplify the purification process.
11.
The crude material is dissolved in EtOAc (20 mL) and then charged onto a silica gel column (diameter = 3 cm, height = 10 cm) of 35 g (80 mL) of silica gel.
12.
The checkers purchased silica gel (acidic) from Kanto Chemical Co., Inc. (40-100 μm). The submitters purchased silica gel (0.040-0.063 mm) from Merck.
13.
The submitters performed GC-MS analysis of the crude material and detected only the peak corresponding to the product, corresponding to the only major spot (R
f = 0.4) on the TLC plate (pentane/ethyl acetate = 8:2, UV detection)
14.
The crude material was dissolved in toluene (10 mL) and then charged onto a column (diameter = 10 cm, height = 11 cm) of 425 g (1000 mL) of silica gel. The column was eluted with
n-hexane/EtOAc = 8:1 (7.0 L) to 2:1 (3.6 L) and 100-mL fractions were collected. Fractions 73-108 were combined.
15.
The major by-product was a dimer of
N,N-diethylbenzamide (R
f = 0.62 (hexane/ethyl acetate = 8:2, UV detection with 254 nm UV lamp), Merck silica gel 60 F
254 plates (0.25 mm, glass-backed) were used for TLC analysis). Fractions 46-70 were combined to obtain 216 mg of such compound.
16.
A very minor condensation may appear eventually in the vacuum line system (< 10 mg). NMR shows it to be exclusively the starting material
N,N-diethylbenzamide. This represents a convenient way to remove trace impurities that may still be present in spite of the silica gel purification.
17.
(E)-N,N-Diethyl-2-styrylbenzamide has the following physicochemical and spectroscopic properties: R
f = 0.4 (pentane/ethyl acetate = 8:2), R
f = 0.34 (hexane/ethyl acetate = 8:2);
1H NMR (400 MHz, CDCl
3) δ: 1.00 (t,
3J = 7.1 Hz, 3 H), 1.31 (t,
3J = 7.1 Hz, 3 H), 3.11 (q,
3J = 7.1 Hz, 2 H), 3.39 (broad s, 1 H), 3.85 (broad s, 1 H), 7.09 (d,
3J = 16.5 Hz, 1 H), 7.13 (d,
3J = 16.5 Hz, 1 H), 7.23-7.47 (m, 8 H), 7.70 (d,
3J = 7.8 Hz, 1 H);
13C NMR (100 MHz, CDCl
3) δ: 12.9 (s, CH
3), 13.8 (s, CH
3), 38.8 (s, CH
2), 42.8 (s, CH
2), 124.9 (s, CH), 125.2 (s, CH), 126.1 (s, CH), 126.5 (s, CH), 127.5 (s, CH), 127.8 (s, CH), 128.6 (s, CH), 128.7 (s, CH), 130.8 (s, CH), 133.6 (s, C
quat), 136.3 (s, C
quat), 137.0 (s, C
quat), 170.3 (s, C
quat); HRMS (ESI):
m/z calc. for [(C
19H
21NO)Na]
+ 302.15208, found 302.15169; IR cm
-1: 2974, 2933, 1626, 1428, 1380, 1363, 1314, 1285, 1220, 1113, 1075, 963, 762, 692, 627, 547, 528; Anal. Calc. for C
19H
21NO: C, 81.68; H, 7.58; N, 5.01. Found: C, 81.29; H, 7.49; N, 4.94.
Handling and Disposal of Hazardous Chemicals
The procedures in this article are intended for use only by persons with prior training in experimental organic chemistry. All hazardous materials should be handled using the standard procedures for work with chemicals described in references such as "Prudent Practices in the Laboratory" (The National Academies Press, Washington, D.C., 2011 www.nap.edu). All chemical waste should be disposed of in accordance with local regulations. For general guidelines for the management of chemical waste, see Chapter 8 of Prudent Practices.
These procedures must be conducted at one's own risk. Organic Syntheses, Inc., its Editors, and its Board of Directors do not warrant or guarantee the safety of individuals using these procedures and hereby disclaim any liability for any injuries or damages claimed to have resulted from or related in any way to the procedures herein.
3. Discussion
The oxidative Heck-type reaction, as pioneered by Fujiwara and Moritani,
2 has emerged as an attractive method for the coupling of arenes and olefins, because in contrast to the traditional Heck reaction,
3 it obviates prior activation of either reaction partner. For this cross-coupling reaction, palladium-based complexes are traditionally privileged catalysts,
4-6 for example in the C-H oxidative olefination of acetanilides with acrylate derivatives reported by de Vries, van Leeuwen et al.,
5 and in the use of remote carboxylic acids as efficient directing groups described by Yu et al.
6 Miura and Satoh et al.,
7 we,
8 and others have looked at other transition metals for these C-H activation processes, specifically rhodium, which often allows lower catalytic loadings, higher selectivities, and broader olefin scope. Whereas acrylates were previously used as the preferred reaction partner, recently we have had some success with the rhodium-catalyzed coupling of unactivated acetanilides (electron-rich arenes) with acrylates, styrenes and even ethylene itself (Scheme 1).
8a
The resulting olefinated products
1 might serve as valuable building blocks. Still, the C-H activation processes of many difficult-to-activate,
electron-poor substrates such as common carbonylated arenes have remained underdeveloped,
9,10 especially those with an (often desired) oxidative character. In addition, we have recently found that benzamides and acetophenones constitute excellent classes of substrates for the rhodium-catalyzed oxidative olefination reaction, resulting in the formation of a diverse set of styrene derivatives
2 and
3.
11 Similarly, vinylic C-H bonds were also utilized in these olefination reactions, providing rapid access to functionalized diene products
4.
12
Previously, we found that tertiary benzamides are substantially more efficient for the
ortho C-H oxidative olefination reaction than primary benzamides such as
5a. Indeed,
2i (the title compound) was isolated in 83% yield (1 mmol scale, 1 mol% Rh catalyst), while
2a, the primary benzamide analogue was only isolated in 60% yield.
11 It seems that the improved electron-richness of the directing group (
tertiary benzamide
5i) was beneficial for the reaction, suggesting that the interaction between the directing group and the Rh catalyst is pivotal, and that it can be tuned with the substitution pattern of the amide (Scheme 2). Furthermore, in the case of unbiased benzamides, that is, when positions
2- and
3- of the arene ring are not protected by a bulky substituent, the di-olefination reaction leading to the undesired product
7 can become an issue. While primary benzamides are already very selective in that respect (only traces of
7a are detected, <10%), tertiary benzamides are found to exclusively form the mono-olefinated product (detection limit < 2%). This we think has also to do with the size of the directing group. Indeed, a large directing group will be less prone to provide the
planar configuration required for an efficient second C-H activation, if it already has a (bulky) styryl substituent on the other
2-position (see
2a,i).
Scheme 1. Rh catalyzed C-H oxidative Heck-type reaction: conditions and isolated yields; selected examples.
8a,11,12 0.5 mol% of [RhCp*Cl
2]
2 and 2 mol% AgSbF
6;
t-AmylOH as solvent. Ratio of coupling partners ranging from 1:1.5 to 1.5:1; run on scales from 0.5 to 1.0 mmol. [a] 2 bar of ethylene. [b] 2.5 mol% of [RhCp*Cl
2]
2 and 10 mol% AgSbF
6. [c] Reaction in 1,4-dioxane. [d] Reaction run at 140 °C. [e] 4.2 equiv of Cu(OAc)
2, 3 equiv of styrene and 2.5 mol% of [RhCp*Cl
2]
2.
Scheme 2. Chemo-selectivity in the Rh catalyzed C-H oxidative Heck-type reaction.
While investigating the suitability of our technique on a preparative 30 mmol scale (Scheme 2), we found it experimentally convenient to increase the reaction concentration (from 0.20 M to 0.74 M) and very importantly to decrease the catalyst loading from 0.5 mol% to 0.25 mol% of the [Cp*RhCl2]2 precatalyst (see experimental procedure). To our surprise, this afforded a substantial improvement of the reaction, as the desired product, (E)-N,N-diethyl-2-styrylbenzamide 2i (the Z stereoisomer was not detected), was obtained in 92% isolated yield.
In conclusion, we have found that tertiary benzamides, such as
N,N-diethylbenzamide, are excellent substrates for the
mono-selective dehydrogenative/oxidative olefination reaction catalyzed by Rh, even at very low catalytic loadings (0.25 mol%). Because of its practicality and chemo-selectivity, we expect that this method will find applications in organic synthesis.
13
Appendix
Chemical Abstracts Nomenclature (Collective Index Number);
(Registry Number)
(E)-N,N-Diethyl-2-styrylbenzamide; (1269637-39-9).
N,N-Diethylbenzamide; (1696-17-9).
Styrene; (100-42-5).
Pentamethylcyclopentadienylrhodium(III) chloride dimer; (12354-85-7).
Silver Hexafluoroantimonate(V); (26042-64-8).
Copper(II) acetate, anhydrous; (142-71-2).
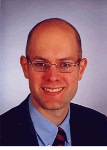 |
Frank Glorius was educated in chemistry at the Universität Hannover, Stanford University (Prof. Paul A. Wender), Max-Planck-Institut für Kohlenforschung and Universität Basel (Prof. Andreas Pfaltz) and Harvard University (Prof. David A. Evans). In 2001 he began his independent research career at the Max-Planck-Institut für Kohlenforschung in Germany (Mentor: Prof. Alois Fürstner). In 2004 he became Assoc. Prof. at the Philipps-Universität Marburg and since 2007 he is a Full Prof. for Organic Chemistry at the Westfälische Wilhelms-Universität Münster, Germany. His research program focuses on the development of new concepts for catalysis and their implementation in organic synthesis. |
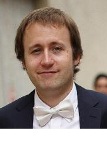 |
Frederic William Patureau was born in 1982 in St. Germain en
Laye, France. He studied biology at the University of Versailles
(France, 2000-2002), and obtained his bachelor's degree in chemistry in 2003 from the University of Paris-Sud Orsay (France). There, he completed his master's degree (2005) in the group of Prof. H. B. Kagan and Prof. J.-C. Fiaud (mentors: Dr. E. Schulz and Dr. M. Mellah). He then moved to the Van't Hoff Institute for Molecular Sciences at the University of Amsterdam (Netherlands), where he obtained his doctoral degree in 2009 (mentor: Prof. J. N. H. Reek). He then joined the group of Prof. F. Glorius at the W.W. University of Münster (Germany), where he became an Alexander von Humboldt Fellow. In November 2011, he started his independent career as Junior Professor of Organometallic Catalysis at the Technische Universität Kaiserslautern. |
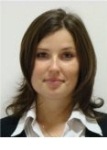 |
Tatiana Besset was educated in chemistry at the University of Grenoble (France) in the group of Dr. Andrew Green, where she obtained her doctoral degree in 2009. She then moved to the Westfälische Wilhelms-Universität Münster (Germany) for a postdoctoral period in the group of Prof. Dr. Frank Glorius. In 2011, she moved to the University of Amsterdam (the Netherlands) in the group of Prof. Dr. Joost N. H. Reek, as postdoctoral fellow. She is currently Assistant Professor (CR2, Chargée de Recherche CNRS) at the University of Rouen, France. |
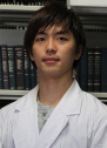 |
Takaaki Harada was born in 1988 in Osaka, Japan. He graduated in 2010 and received his M. S. degree in 2012 from the University of Tokyo. The same year he started his Ph. D. study under the supervision of Professor Tohru Fukuyama. His current interest is enantioselective total synthesis of complex natural products. |
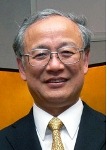 |
Tohru Fukuyama received his Ph.D. in 1977 from Harvard University with Yoshito Kishi. He remained in Kishi's group as a postdoctoral fellow until 1978 when he was appointed as Assistant Professor of Chemistry at Rice University. After seventeen years on the faculty at Rice, he returned to his home country and joined the faculty of the University of Tokyo in 1995, where he is currently Professor of Pharmaceutical Sciences. He has primarily been involved in the total synthesis of complex natural products of biological and medicinal importance. He often chooses target molecules that require development of new concepts in synthetic design and/or new methodology for their total synthesis. |
Copyright © 1921-, Organic Syntheses, Inc. All Rights Reserved