Org. Synth. 2013, 90, 87-95
DOI: 10.15227/orgsyn.090.0087
Intermolecular retro-Cope Type Hydroxylamination of Alkynes with NH2OH: E-1-(1-Hydroxycyclohexyl)ethanone oxime
Submitted by Francis Loiseau and André M. Beauchemin
1.
Checked by Nikolas Huwyler and Erick M. Carreira.
1. Procedure
A. E-1-(1-Hydroxycyclohexyl)ethanone oxime. A 100-mL one-necked (TS 14/23) round-bottomed flask is successively charged with 1-ethynylcyclohexanol (5.00 g, 40.3 mmol, 1 equiv) (Notes 1 and 2), 2-methyl-1-propanol (40 mL) (Note 3) and aqueous hydroxylamine (Note 4), (50 wt. %, 6.18 mL, 101 mmol, 2.5 equiv). The flask is equipped with a Teflon-coated magnetic stir bar (6 × 15 mm) and a reflux condenser, which is sealed with a rubber septum. Argon is introduced by means of a syringe needle through the septum and the system is flushed with argon for 5 min before the flask is immersed in an oil-bath at 125 °C (Note 5). The reaction mixture is refluxed for 18 h under an atmosphere of argon (Note 6). The solution stays clear and colorless for the duration of the reaction. After cooling to ambient temperature, the reaction mixture is transferred to a 250 mL round-bottomed flask and concentrated by rotary evaporation (45 °C, 25 mmHg). The resulting oil is azeotroped twice by adding hot toluene (2 x 50 mL) and concentrating by rotary evaporation (45 °C, 25 mmHg) (Note 7). The crude white solid (6.36-6.42 g) is transferred to a 50-mL Erlenmeyer flask and dissolved in 18 mL of near-boiling 1,2-dichloroethane (80 °C). The resulting solution is left to cool to ambient temperature over 2 h, allowing crystallization to occur, and is further cooled at 0 °C for 2 h. The resulting crystals are collected by suction filtration on a Büchner funnel, and suction-dried for 30 min. The crystals are rinsed with 5 mL of ice-cold hexanes, suction-dried again for 30 min, transferred to a 50-mL round-bottomed flask and dried for 1 h at 0.1 mmHg at ambient temperature to provide E-1-(1-hydroxycyclohexyl)-ethanone oxime as white needle crystals (5.27-5.42 g, 83-86% yield) (Notes 8 and 9).
2. Notes
1.
The submitters used the following materials as received: 1-ethynylcyclohexanol (≥99%, Sigma-Aldrich), 2-methyl-1-propanol (ACS reagent grade, ≥99%, GFS Chemicals), toluene (ACS grade, 99.9%, Fisher Scientific), 1,2-dichloroethane (ACS grade, 99.9%, Fisher Scientific) and hexanes (ACS grade, 99.9%, Fisher Scientific).
2.
The checkers obtained 2-methyl-1-propanol (ACS grade, ≥99.0%), 1-ethynylcyclohexanol (≥99%), and hexane (HPLC grade, ≥95%) from Sigma-Aldrich Co., while toluene (ACS grade, ≥99.7%) and 1,2-dichloroethane (ACS grade, ≥99.5%) were purchased from Fluka. All reagents and solvents were used as received.
3.
2-Methyl-1-propanol was chosen as a solvent for the reaction because its boiling point was in the temperature range needed for the desired reactivity and reaction times. 1-Propanol can be alternatively used as a solvent, using microwave heating (4 h, 140 °C) or heating in a sealed tube placed in an oil bath (18 h, 110 °C), yielding similar results.
4.
Aqueous hydroxylamine (50 wt. %) was purchased from Aldrich Chemical Co. and used as received.
Caution: Due to the high energy content of NH2OH, appropriate care should be taken when conducting these experiments. The hydroxylamine concentration should not be increased beyond 5-10 wt% (i.e. the typical reaction conditions) and appropriate safety controls should be performed before scaling up this chemistry above the gram-scale, especially at very high temperatures. Note: NH
2OH has been used on the 2 mol scale under similar conditions (reflux in EtOH) in a previously reported procedure. (Steiger, R. E.
Org. Synth., Coll. Vol. III,
1955, 91).
5.
The success of the reaction does not depend on having previously dried the glassware or on adding the reagents under an inert atmosphere.
6.
The progress of the reaction can be followed by TLC analysis on silica gel with 30% EtOAc-hexane as eluent and visualized with potassium permanganate, ensuring proper evaporation of the reaction solvent by heating the plate pre-elution. The propargylic alcohol starting material has R
f = 0.55 and the α-hydroxyoxime product has R
f = 0.37.
7.
The high H-bonding affinity of the oxime product causes binding to alcoholic solvents. Toluene is used to azeotropically distill off the remaining 2-methyl-1-propanol. In cases when the oxime is obtained as a solid after the initial or second concentration, the mixture, after addition of hot toluene, can be heated and swirled until complete dissolution before concentrating again. (The crude oxime melts below the boiling point of toluene and is miscible in the solvent when in the liquid state).
8.
The product displayed the following spectroscopic and physiochemical properties: mp 101-103 °C;
1H NMR
pdf (400 MHz, CDCl
3) δ: 1.14-1.30 (m, 1 H), 1.48-1.77 (m, 9 H), 1.90 (s, 3 H), 2.85 (br s, 1 H), 8.44 (br s, 1 H);
13C NMR
pdf (101 MHz, CDCl
3) δ: 9.7, 21.4, 25.4, 35.0, 73.7, 162.4; IR (film) cm
-1: 3460, 3202, 3147, 2932, 2852, 1466, 1456, 1445, 1431, 1420, 1371, 1312, 1258, 1177, 1156, 1132, 1068, 1054, 998, 974, 936, 884, 846, 748; HRMS (EI)
m/z: [M]
+ calcd for C
7H
15NO
2: 157.1098. Found: 157.1104;
m/z: [M-OH]
+ for C
7H
12NO: 140.1070. Found: 140.1072; Elemental anal. calcd for C
7H
15NO
2: C, 61.12; H, 9.62; N, 8.91. Found: C, 61.08; H, 9.53; N, 8.91.
9.
The product was found to be stable upon storage for at least 2 weeks at room temperature and ambient air.
Handling and Disposal of Hazardous Chemicals
The procedures in this article are intended for use only by persons with prior training in experimental organic chemistry. All hazardous materials should be handled using the standard procedures for work with chemicals described in references such as "Prudent Practices in the Laboratory" (The National Academies Press, Washington, D.C., 2011 www.nap.edu). All chemical waste should be disposed of in accordance with local regulations. For general guidelines for the management of chemical waste, see Chapter 8 of Prudent Practices.
These procedures must be conducted at one's own risk. Organic Syntheses, Inc., its Editors, and its Board of Directors do not warrant or guarantee the safety of individuals using these procedures and hereby disclaim any liability for any injuries or damages claimed to have resulted from or related in any way to the procedures herein.
3. Discussion
Oximes are useful building blocks or key intermediates for a variety of organic transformations, including the Beckman rearrangement (yielding amides), the Neber reaction (yielding α-amino carbonyl moieties), and in diverse amination strategies with applications ranging from amination of organometallic reagents (electrophilic amination) to heterocyclic synthesis (via electrophilic, nucleophilic, radical-based or amino-Heck amination manifolds).
2 O-Protected oximes are also good electrophiles providing access to amine derivatives upon reaction with various nucleophiles, and stereoselective variants have been investigated.
3 As such, oximes provide access to various nitrogen-containing functional groups that are prevalent in medicinal chemistry and in natural products.
A variety of approaches are available to access oximes efficiently. The simple condensation of carbonyl compounds and NH
2OH (typically formed from its salt NH
2OH•HCl and a mild base such as NaOAc) is often simple, efficient and practical.
4 However, several routes have been developed to complement this method, which are especially useful when the desired carbonyl compound is not readily available or when alternative starting materials are commercially or more readily available. Such routes include (1) the direct oxidation of primary amines to oximes;
5 (2) the reduction of nitroalkanes or nitroalkenes;
6 (3) a 2-step Mitsunobu approach on primary and secondary alcohols;
7 and (4) the nitrosation reactivity of alkenes.
8
Recently, numerous important developments have extended the synthetic reach of alkene and alkyne hydroamination to a variety of nitrogen-containing molecules.
9 In particular, metal-catalyzed alkyne hydroamination is emerging as a direct approach to a variety of imine derivatives, through intra- and intermolecular reactions.
10 As part of efforts to enable oxime formation using alkyne hydroamination, and to develop the use of hydroxylamines and hydrazines in metal-free Cope-type hydroaminations,
11 we previously reported that oximes are formed directly upon heating alkynes and NH
2OH (eq 1).
12,13
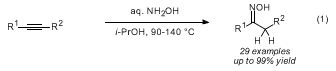
After several rounds of reaction optimization, we developed several conditions that allow both terminal and internal alkynes to afford the Markovnikov hydroamination products effectively. The original conditions (Table 1, conditions A) involve conventional heating done in sealed tubes with 1,4-dioxane used as the solvent, and an excess of aqueous hydroxylamine (2.5 equiv). Optimized and preferred reaction conditions (conditions B) feature the use of a microwave reactor, which allows slightly increased yields and significantly shorter reaction times (<10 h), and use alcoholic solvents.
The present procedure describes one representative example for the synthesis of oximes from alkynes and NH
2OH, and produces
E-1-(1-hydroxycyclohexyl)ethanone oxime (>5 g) in greater than 83 % yield. The procedure uses a simple reflux in 2-methyl-1-propanol (
conditions C) and is complementary to our previously published conditions. Further modifications can be necessary in some cases when product degradation occurs under standard reflux conditions (notably when using the propargylamine-based substrates). These last reaction conditions (
conditions D) are milder, involve conventional reflux for a longer reaction time at a lower temperature, and feature the use of a reduced excess of aqueous hydroxylamine (1.5 equiv rather than 2.5 equiv, as reaction optimization revealed that the reaction is higher yielding under those conditions).
14
As shown in Table 1, the reaction is compatible with a variety of alkynes (aromatic, aliphatic, enynes, sterically hindered), standard protecting groups, free hydroxyl groups, basic nitrogen atoms (
sp2 and
sp3), and amides. Internal alkynes can also be reacted, albeit resulting in reduced yields. In most cases, the unreacted starting alkynes can be recovered, and the products can be conveniently isolated by recrystallization or chromatography.
Table 1. Synthesis of Oximes from the Cope-type Hydroamination of Alkynes with NH2OH
The reaction is most efficient when protic solvents (such as 1-propanol, 2-propanol or 2-methylpropan-1-ol) are used. The increased reactivity observed under these conditions is consistent with such alcohols both stabilizing the expected enamine N-oxide intermediate (I) and facilitating the proton transfer step required to access a neutral N-hydroxyenamine (III), that can subsequently tautomerize to the oxime product (IV). Computational results support a bimolecular proton transfer pathway in which the alcohol mediates the proton transfer through a five-membered, cyclic transition state (II), over an intramolecular proton transfer or stepwise alternatives.
Scheme 1
Appendix
Chemical Abstracts Nomenclature (Collective Index Number);
(Registry Number)
1-Ethynylcyclohexanol; (78-27-3)
Hydroxylamine; (7803-49-8)
E-1-(1-Hydroxycyclohexyl)ethanone oxime; (62114-93-6)
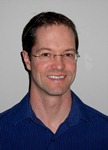 |
André M. Beauchemin grew up in Québec city and completed his B.Sc. in 1996 from Université Laval. He pursued his Ph.D. under the guidance of André B. Charette at the Université de Montréal and worked as a NSERC postdoctoral fellow with Prof. David A. Evans at Harvard University. He began his independent career at the University of Ottawa in August 2004. His research group is interested in novel methods and concepts for accessing nitrogen-containing molecules, and has developed a metal-free amination portfolio for the synthesis of hydroxylamines, hydrazines, oximes, saturated nitrogen heterocycles, pyridines, pyrazines, β-aminocarbonyl motifs and enantioenriched diamines. |
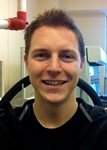 |
Francis Loiseau was born and raised in Montreal, Canada, and received his B.Sc. degree in Chemistry from McGill University in 2005, where he performed undergraduate research in the laboratories of James L. Gleason and Mark P. Andrews. He went on to work for two years at Handy Chemicals in Candiac, Canada, where he worked at the synthesis of concrete and paint additives. He is currently a FQRNT Fellow at the University of Ottawa pursuing a Ph. D. degree under the direction of Professor André Beauchemin. His research focuses on the development of metal-free Cope-type hydroamination. |
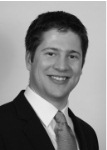 |
Nikolas Huwyler was born and raised in Zurich, Switzerland, and obtained a M.Sc. degree in Chemistry from ETH Zurich in 2009. During his undergraduate education he performed research in the laboratories of Prof. Erick M. Carreira and Prof. Antonio Togni at the ETH Zurich and completed his studies with a Master's thesis in the laboratories of Prof. Timothy F. Jamison at Massachusetts Institute of Technology, Cambridge MA, USA, where he had a 6 month stay as a visiting scientist. He is currently pursuing doctoral studies in synthetic organic chemistry with Prof. Carreira focusing on the total synthesis of natural products. |
Copyright © 1921-, Organic Syntheses, Inc. All Rights Reserved