Org. Synth. 2008, 85, 179
DOI: 10.15227/orgsyn.085.0179
REGIOSELECTIVE SYNTHESIS OF 1,3,5-TRISUBSTITUTED PYRAZOLES BY THE REACTION OF N-MONOSUBSTITUTED HYDRAZONES WITH NITROOLEFINS
Submitted by Xiaohu Deng and Neelakandha S. Mani
1.
Checked by Kay M. Brummond, Daitao Chen, and Josh Osbourn
2.
1. Procedure
A. 5-Benzo[1,3]dioxol-5-yl-3-(4-chlorophenyl)-1-methyl-1H-pyrazole (2). A 500-mL, three-necked, round-bottomed flask equipped with a mechanical stirrer (fitted with a 7.5-cm glass paddle), a condenser (open to the atmosphere) and a rubber septum is charged with 4-chlorobenzaldehyde (5.00 g, 35.5 mmol, 1.25 equiv) and MeOH (200 mL) (Note 1). Methylhydrazine (1.9 mL, 1.66 g, 35.5 mmol, 1.25 equiv) is added to this solution dropwise via syringe over 3 min (Note 1). After 2 h at room temperature, the formation of hydrazone 1 is complete (Note 2). The septum is removed and 3,4-methylenedioxy-β-nitrostyrene (5.50 g, 28.5 mmol) is added as a solid in one portion (Note 3). The reaction mixture is stirred at room temperature open to air for 72 h (Notes 4–6). The condenser is replaced with a 125-mL, pressure-equalizing addition funnel and water (60 mL) is added to the mixture over 20 min. The resulting suspension is stirred at room temperature for an additional 1 h. The yellow solid that is formed is collected on a 250-mL fritted glass Büchner funnel by vacuum filtration (washing with ca. 30 mL of 1:1 MeOH/H2O) and is suction-dried overnight. The yellow solid is dissolved in a minimum amount of boiling MeOH, then after being cooled to room temperature, the product is collected by suction filtration in 125-mL fritted glass Büchner funnel as a fine brown powder (6.07 g, 19.3 mmol, 68%) (Note 7).
B. 1-Benzyl-3-(4-chlorophenyl)-5-p-tolyl-1H-pyrazole (4). In a 500-mL, one-necked, round-bottomed flask equipped with a 3.8-cm, egg-shaped Teflon-coated magnetic stirring bar and a condenser, 4-chlorobenzaldehyde (4.86 g, 34.5 mmol, 1.25 equiv) is dissolved in MeOH (150 mL) and then water (10 mL) is added (Note 8). Benzylhydrazine dihydrochloride (6.75 g, 34.5 mmol, 1.25 equiv) is added in one portion (Note 9). After stirring the mixture at room temperature for 3 h, hydrazone 3 is formed (Note 10). 4-Methyl-β-nitrostyrene (4.51 g, 27.7 mmol) (Note 11) is added in one portion and the reaction solution is stirred at room temperature open to air until complete (Notes 12, 13). The condenser is replaced with a 125-mL, pressure-equalizing addition funnel and water (50 mL) is slowly added into the mixture over 20 min and the resulting white suspension is stirred at room temperature for an additional 1 h. The resulting white solid is collected on a 250-mL fritted glass Büchner funnel by vacuum filtration (washing with ca. 30 mL of 1:1 MeOH/H2O) and is suction-dried (ca. 10 min). The solid is transferred into a 50-mL, round-bottomed flask and further dried under vacuum at room temperature for 8 h. The title compound is obtained as a white solid (9.12 g, 25.3 mmol, 92%) (Note 14).
2. Notes
1.
4-Chlorobenzaldehyde (97% purity) and methylhydrazine (98% purity) were purchased from Aldrich. Methanol (HPLC grade) was purchased from EMD. All reagents were used as received without further purification. HPLC
tR = 8.68 min
(Note 2) for 4-chlorobenzaldehyde.
2.
The checkers monitored the progress of the reaction by
1H NMR spectroscopy. (Typical procedure for
1H NMR analysis: an aliquot of the reaction mixture was transferred into an NMR tube via pipette. The NMR tube was fitted with a rubber septum, connected to a vacuum with a needle, the solvent was evaporated and the residue was dissolved in CDCl
3). NMR data for hydrazone
1; 1H NMR
pdf (500 MHz, CDCl
3) δ: 3.01 (s, 3 H), 7.34 (d,
J = 8.5 Hz, 2 H), 7.49 (s, 1 H), 7.52 (d,
J = 8.5 Hz, 2 H). The submitters monitored the formation of hydrazone
1 by HPLC analysis. All HPLC analyses were performed on a Hewlett Packard 1100 (Agilent ZORBAX® Eclipse XDB-C8, 5 µm, 4.6 × 150 mm, 1 mL/min, acetonitrile/water with 0.05% trifluoroacetic acid : 1% acetonitrile/99% water to 99% acetonitrile/1% water ramp over 8 min, then hold at 99% acetonitrile/1% water) HPLC
tR = 7.43 min for
1.
3.
3,4-Methylenedioxy-β-nitrostyrene (98% purity) was purchased from Alfa Aesar and used as received. HPLC
tR = 8.83 min
(Note 2).
4.
Air is essential for the reaction to proceed.
5.
The reaction time is dependent upon the scale and the stirring speed.
1H NMR analysis of small aliquots is desirable to follow the reaction progress. The submitters reported a reaction time of 40 h for a 5 g reaction; the checkers observe a reaction time of 72 h for the same reaction scale.
6.
As the reaction proceeds 3,4-methylenedioxy-β-nitrostyrene slowly dissolves and the product precipitates, evidenced by a brown heterogeneous mixture turning homogeneous and a yellow precipitate forming after 1-2 h.
7.
The product displayed the following physicochemical properties: mp 117–120 °C;
1H NMR
pdf (500 MHz, CDCl
3) δ: 3.90 (s, 3 H), 6.05 (s, 2 H), 6.52 (s, 1 H), 6.91-6.92 (m, 3 H), 7.37 (dt,
J = 9.0, 2.0 Hz, 2 H), 7.75 (dt,
J = 9.0, 2.0 Hz, 2 H);
13C NMR
pdf (125.7 MHz, CDCl
3) δ: 37.7, 101.7, 103.3, 108.8, 109.4, 122.9, 124.3, 126.9, 129.0, 132.2, 133.5, 145.1, 148.1, 148.2, 149.5; IR (film) cm
−1: 2895 (w), 1554 (w), 1478 (s); MS
m/z (relative intensity): 312 (M
+, 100%); HRMS-ESI
m/z: [M]
+ calcd for C
17H
13ClN
2O
2 312.0666; found, 312.0663. Anal. Calcd for C
17H
13ClN
2O
2:
C, 65.29; H, 4.19; N, 8.96. Found: C, 65.09; H, 4.13; N, 9.00. The submitters reported HPLC
tR = 10.47 min. The crude pyrazole (prior to recrystallization) is contaminated with a small amount of the 1,4-addition product (
E)-1-(1-(benzo[d][1,3]dioxol-5-yl)-2-nitroethyl)-2-(4-chlorobenzylidene)-1-methylhydrazine as evidenced by the three resonances: 5.41 (dd,
J = 13.2, 9.5 Hz, 1 H), 4.98 (dd,
J = 9.5, 4.8 Hz, 1 H), 4.60 (dd,
J = 13.2, 4.8, 1 H). For complete characterization of the 1,4-addition product see the original disclosure of this procedure by the authors.
3
8.
Water (distilled) is necessary for hydrazone and pyrazole formation when the HCl salt of the hydrazine is used.
9.
Benzylhydrazine dihydrochloride (97% purity) was purchased from Aldrich and used as received.
10.
The checkers monitored the progress of the reaction by
1H NMR spectroscopy. (Typical procedure for
1H NMR analysis: an aliquot of the reaction mixture was transferred into an NMR tube via pipette. The NMR tube was fitted with a rubber septum, connected to a vacuum with a needle, the solvent was evaporated and the residue was dissolved in CDCl
3.) NMR data for hydrazone
3; 1H NMR
pdf (500 MHz, CDCl
3) δ: 4.44 (s, 2 H), 5.74 (br s, 1 H), 7.30 (d,
J = 8.5 Hz, 2 H), 7.32-7.28 (m, 1 H), 7.36-7.37 (m, 4 H), 7.48 (d,
J = 8.5 Hz, 2 H), 7.55 (s, 1 H). The submitters reported the HPLC
tR = 10.05 min for
3 (Note 2).
11.
4-Methyl-β-nitrostyrene (98% purity) was purchased from Alfa Aesar and used as received. HPLC
tR = 9.42 min
(Note 2).
12.
Whereas the submitters report a 48 h reaction time, the checkers observed a reaction time of 88 to 92 h. The progress of the reaction was monitored by
1H NMR spectroscopy.
13.
As the reaction proceeds the product precipitates as a white solid after ca. 1-2 h.
14.
No purification is necessary for 1-benzyl-3-(4-chlorophenyl)-5-
p-tolyl-1
H-pyrazole (
4). The product displayed the following physicochemical properties:: mp 132-133 °C; HPLC
tR =11.99 min
(Note 2); R
f = 0.43 (hexanes/EtOAc, 4/1);
1H NMR (500 MHz, CDCl
3) δ: 2.40 (s, 3 H), 5.38 (s, 2 H), 6.61 (s, 1 H), 7.11 (d,
J = 7.0 Hz, 2 H), 7.21-7.32 (m, 7 H), 7.38 (dt,
J = 9.0, 2.0 Hz, 2 H), 7.80 (dt,
J = 9.0, 2.0 Hz, 2 H);
13C NMR
pdf (125.7 MHz, CD
2Cl
2) δ: 21.5, 53.4, 103.7, 126.9, 127.1, 127.6, 127.7, 128.8, 129.0 (2C), 129.6, 132.3, 133.5, 137.9, 139.0, 146.0, 150.1; IR (film) cm
−1: 3028 (w), 2929 (w), 1490 (w), 1436 (s); MS
m/z (relative intensity): 358 (M
+, 80%), 239 (26%), 91 (100%); HRMS-ESI
m/z: [M]
+ calcd for C
23H
19ClN
2 358.1237; found, 358.1225; Anal. Calcd for C
23H
19ClN
2, C, 76.98; H, 5.34; N, 7.81. Found: C, 76.85; H, 5.34; N, 7.84. The submitters reported greater than 98% purity based upon HPLC analysis and
tR = 11.99 min
(Note 2).
Handling and Disposal of Hazardous Chemicals
The procedures in this article are intended for use only by persons with prior training in experimental organic chemistry. All hazardous materials should be handled using the standard procedures for work with chemicals described in references such as "Prudent Practices in the Laboratory" (The National Academies Press, Washington, D.C., 2011 www.nap.edu). All chemical waste should be disposed of in accordance with local regulations. For general guidelines for the management of chemical waste, see Chapter 8 of Prudent Practices.
These procedures must be conducted at one's own risk. Organic Syntheses, Inc., its Editors, and its Board of Directors do not warrant or guarantee the safety of individuals using these procedures and hereby disclaim any liability for any injuries or damages claimed to have resulted from or related in any way to the procedures herein.
3. Discussion
Substituted pyrazoles are important synthetic targets in the pharmaceutical industry.
4 Regioselective synthesis of the pyrazole ring remains a significant challenge for organic chemists.
5 Inspired by the literature precedent reactions of hydrazones and nitroolefins
6 we developed a general, one-pot, regioselective synthesis of substituted pyrazoles from
N-monosubstituted hydrazones and nitro-olefins.
The outcome of the reaction depended upon the nature of solvents used (Table 1). Non-polar solvents such as toluene are not a good choice for the reaction, with no reaction being observed. Aprotic polar solvents generally favored the formation of Michael addition product 3, with the exception of CH2Cl2. Protic polar solvents favored the formation of pyrazole 2. Although AcOH and CH2Cl2 also afforded high 2:3 ratios, the reaction in alcoholic solvents such as MeOH and EtOH was much cleaner and provided the best yields of 2.
Table 1. Solvent Effect on the Pyrazole Formation Reaction.
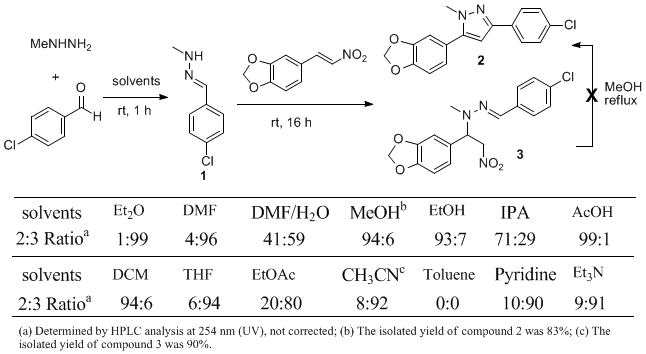
This pyrazole formation reaction is fairly general with nitroolefins bearing various substituents at the R3 and R4 positions (Table 2). Steric effects at R2 position play a small role; for example, sterically congested 2,2-dimethylpropionaldehyde affords pyrazole 7 in 56% yield (Entry 5). In contrast, electronic effects are prominent. Whereas electron-donating or slightly electron-withdrawing aldehydes provide pyrazole products smoothly, electron-demanding 4-nitrobenzaldehyde gives the Michael addition product 9 in 90% yield (Entry 7), which does not cyclize even at reflux temperature. At the R1 position, both electronic and steric effects are important. The pyrazole formation reaction proceeds smoothly with primary alkyl hydrazones such as methyl hydrazones (Entries 1-7) and benzyl hydrazone (Entry 8). However, as the bulk of the R1 group increases, the reaction slows or requires higher temperature. For example, with isopropylhydrazine, the pyrazole formation reaction was not complete after 7 days at room temperature with only 26% isolated yield (Entry 9). In the case of tert-butylhydrazine, after refluxing for 4 days, a different 1-t-butyl-3,4-diarylpyrazole isomer 12 was isolated in 15% yield (Entry 10). An electronic effect related to the hydrazone substituents is apparent with electron-deficient phenyl hydrazines. In entry 11, reflux temperature was required for 4 days to give 42% yield of pyrazole 13. Interestingly, this effect can be moderated through the use of an electron-donating aldehyde such as propionaldehyde, which gives a 76% yield of pyrazole 14 at room temperature (Entry 12).
Table 2. Three-component Reaction for Pyrazole Synthesis
A possible reaction pathway has been proposed, as shown in Scheme 1. The first step is a reversible cycloaddition to give a 4-nitro-pyrazolidine intermediate, probably proceeding through a 1,3-dipole intermediate generated
in situ, as proposed in literature on the reaction of hydrazones with other electron-deficient olefins.
7 This process is in competition with an irreversible Michael addition process.
8 In one case, the key intermediate was directly observed on NMR experiments and was fully characterized. The key pyrazolidine intermediate then undergoes a slow oxidation by air, followed by a fast elimination of HNO
29 to afford the pyrazole product.
Scheme 1. Proposed Mechanism
Appendix
Chemical Abstracts Nomenclature (Collective Index Number);
(Registry Number)
4-Chlorobenzaldehyde; (104-88-1)
Methylhydrazine; (60-34-4)
Benzylhydrazine dihydrochloride; (20570-96-1)
(E)-3,4-Methylenedioxy-β-nitrostyrene; (22568-48-5)
trans-p-Methyl-β-nitrostyrene:
Benzene, 1-methyl-4-[(1E)-2-nitroethenyl]-; (5153-68-4)
5-Benzo[1,3]dioxol-5-yl-3-(4-chloro-phenyl)-1-methyl-1H-pyrazole:
1H-Pyrazole, 5-(1,3-benzodioxol-5-yl)-3-(4-chlorophenyl)-1-methyl-; (908329-89-5)
1-Benzyl-3-(4-chloro-phenyl)-5-p-tolyl-1H-pyrazole:
1H-Pyrazole, 3-(4-chlorophenyl)-5-(4-methylphenyl)-1-(phenylmethyl)-; (908329-95-3)
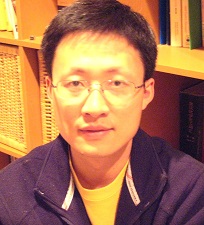 |
Xiaohu Deng was born in 1974 in Jingdezhen, China. He received a B.S. and a M.S. degree in chemistry under the supervision of Prof. Zi Gao from Fudan University in 1995 and 1997, respectively. After completing his doctoral dissertation in 2001 with Prof. Lanny S. Liebeskind at Emory University, he has been working in Johnson & Johnson Pharmaceutical R&D LLC in San Diego since. His research focuses on the design and development of practical synthetic methods to drug candidates.
|
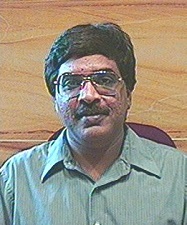 |
Neelakandha Mani was born in 1957 in Kozhikode, India. He completed his Bachelors in Chemistry at Malabar Christian College and Ph.D. at Indian Institute of Science Bangalore with G.S.R. Subba Rao. He spent 3 years with Anthony G.M. Barrett (Northwestern and Colorado State University) and 4 years with Craig A. Townsend (Johns Hopkins University) as post-doctoral researcher. After 5 years as a scientist at Council of Scientific and Industrial Research (CSIR), India and 3 years as a senior scientist at Ligand Pharmaceuticals he moved to Johnson & Johnson in 2000. His research interests include synthesis of heterocycles, design of practical routes to drug molecules and development of environmentally friendly synthetic methods.
|
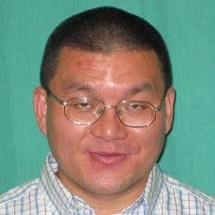 |
Daitao Chen was born in Sichuan, P.R. China in 1974. He received his B.Sc. in 1996 and M.S. in 1999 at Peking University. He then moved to Emory University and obtained his Ph.D. in 2004 under the guidance of Dr. Debra Mohler. After working as a post-doctoral researcher in Dr. Kay Brummond's group at the University of Pittsburgh, he joined Scynexis, Inc (RTP, NC) in 2007. He is currently working on the DNDi team as a research scientist.
|
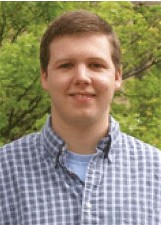 |
Josh Osbourn was born in 1985 in Shinnston, West Virginia. In 2007, he graduated from West Virginia University with a B.S. degree in chemistry. During his time at WVU, he worked in the laboratory of Professor George O'Doherty and was supported through a Pfizer undergraduate research fellowship. In 2007, he entered the Ph.D. program at the University of Pittsburgh and is currently pursuing graduate studies in the laboratory of Professor Kay Brummond.
|
Copyright © 1921-, Organic Syntheses, Inc. All Rights Reserved