Org. Synth. 2010, 87, 1-2
DOI: 10.15227/orgsyn.087.0001
METAL-FREE ONE-POT OXIDATIVE AMINATION OF AROMATIC ALDEHYDES: CONVERSION OF BENZALDEHYDE TO N-BENZOYL PYRROLIDINE
Submitted by Kekeli Ekoue-Kovi and Christian Wolf
1.
Checked by Vikram Bhat and Viresh Rawal.
1. Procedure
Caution! Reactions and subsequent operations involving peracids and peroxy compounds should be run behind a safety shield. Peroxy compounds should be added to the organic material, never the reverse. New or unfamiliar reactions, particularly those run at elevated temperatures, should be run first on a small scale. Highly concentrated solutions of TBHP are potentially hazardous and can undergo violent decomposition upon exposure to certain metal salts. Strong acids must never be added to high-strength TBHP solutions. Highly concentrated solutions of TBHP are best stored in high-density polyethylene bottles rather than glass because of the potential for pressure buildup due to decomposition forming oxygen gas. For additional discussion, see Gao, Y.; Hanson, R. M.; Klunder, J. M.; Ko, S. Y.; Masamune, H.; Sharpless, K. B. J. Am. Chem. Soc. 1987, 109, 5765-5780. [Note added November 2011].
A 250-mL two-necked, round-bottomed flask equipped with a stirring bar, a septum, and a reflux condenser fitted with a nitrogen adapter connected to a dual manifold is charged with nitrogen. The flask is immersed in an oil bath equipped with an external thermometer. An inert atmosphere is maintained during the course of the reaction. Anhydrous acetonitrile (50 mL) (Note 1) is added through the rubber septum via the repeated use of a 20-mL syringe. Benzaldehyde (5.71 mL, 6.0 g, 57 mmol, 1.0 equiv) (Note 2) is then added through the rubber septum using both a five-mL and a one-mL syringe. Pyrrolidine (5.66 mL, 4.82 g, 68 mmol, 1.0 equiv) (Note 3) is added next by a five-mL syringe followed by a solution of tert-butyl hydroperoxide in decane (12.4 mL, 62-74 mmol, 1.09-1.30 equiv) (Notes 4 and 5) using a 10-mL syringe. While maintaining a positive nitrogen pressure, the rubber septum is replaced with a glass stopper. After stirring for 5 min, the yellow reaction mixture is heated in the oil bath to 70 °C for 6 h, and the reaction progress is monitored by TLC (Notes 6 and 7). Upon complete consumption of benzaldehyde, the flask is removed from the oil bath and the reaction mixture cooled to room temperature. The reaction mixture is tested for the presence of peroxides using starch-KI paper, which indicated the absence of peroxides. The solution is then diluted with 100 mL of water and transferred to a 500-mL round-bottomed flask. The reaction flask is rinsed with dichloromethane (2 × 10 mL), and the rinses are transferred to the 500-mL flask. The resulting mixture is concentrated to approximately two-thirds of its initial volume by rotary evaporation (25 °C, 30 mmHg). The concentrated solution is then transferred to a 250-mL separatory funnel and extracted with dichloromethane (4 × 40 mL). The combined organic phases are washed with brine, dried over MgSO4 (7 g), filtered and concentrated by rotary evaporation (25 °C, 30 mmHg) to afford an orange oil. The crude product is purified by flash chromatography (Note 8) using a glass column and flash-grade silica gel. The column is eluted with a total of 3.5 L of ethyl acetate:hexanes (1.2:1) and the fractions containing the desired product are combined and concentrated by rotary evaporation (25 °C, 30 mmHg) and then under high vacuum (25 °C, 1mmHg) for 2 h to give 8.74 g (49.9 mmol, 88%) of N-benzoyl pyrrolidine as a light yellow oil (Note 9).
2. Notes
1.
Anhydrous acetonitrile (99.8%, Cat. No. 271004) was obtained from Sigma-Aldrich, Inc. and used as received.
2.
Benzaldehyde (99.5%, Cat. No. 418099) was obtained from Sigma-Aldrich, Inc. and used as received.
3.
Checkers obtained
pyrrolidine (99%, Cat. No. P73803) from Sigma-Aldrich, Inc. and used it as received. Submitters purchased
pyrrolidine (99%, Cat. No. 13208-2500) from Acros, Inc. and employed it without further purification.
4.
tert-Butyl hydroperoxide (5-6 M in decane, Cat. No. 416665) was obtained from Sigma-Aldrich, Inc. and used as received.
5.
The reaction was carried out in duplicate on a 6.0 g scale.
6.
Checkers carried out TLC analysis using Whatman 60 Å glass plates with ethyl acetate:hexanes (1.2:1) as the eluent and UV lamp (254 nm) for visualization. The R
f values of benzaldehyde and the product (
N-benzoyl pyrrolidine) were found to be 0.9 and 0.3, respectively. Submitters conducted TLC on silica gel 60 plates (Fisher Scientific) using dichloromethane as mobile phase and a UV lamp (254 nm) for visualization. The R
f of benzaldehyde and the product (
N-benzoyl pyrrolidine) were determined as 0.9 and 0.5, respectively.
7.
Submitters also followed the reaction by GC/MS: Varian GC Mass Saturn 2100T spectrometer using a Varian FactorFour capillary column VF-5ms (30 m × 0.25 mm) coated with 5% phenylpolysiloxane and 95% dimethylpolysiloxane. The oven temperature was held at 80 °C for 2 min and then increased to 260 °C over 14 min. The product was eluted after 10.7 min and detected by EI/MS.
8.
Checkers performed flash chromatography on Kieselgel 60, particle size 0.032-0.063 mm using 3.5 L ethyl acetate:hexanes (1.2:1) as mobile phase. A glass column (8-cm diameter) was packed with a slurry of 300 g silica gel (18 cm height). Fractions of 25 mL were collected from the column and analyzed by TLC with UV detection as described above. Desired product was found in fractions 40-118. Submitters used dichloromethane:ethyl acetate:hexanes (17.5:4:1) as mobile phase. The product has an R
f of 0.3 with this mobile phase. The fractions were collected using 20 mL test tubes. Elution of the product started after 700 mL of the mobile phase was collected and the chromatographic process was continued with 2.5 L of the same mobile phase.
9.
N-Benzoyl pyrrolidine can be stored under air atmosphere at room temperature. Checkers found that during storage under ambient conditions, the color of the product deepens to reddish-amber with no change in the NMR or HRMS: UV-Vis (dichloromethane, 1.57 μg/mL) λ
max 230.1 nm; IR (neat) 2971, 2874, 1626, 1576, 1446, 1419 cm
-1;
1H NMR
pdf (500 MHz, CDCl
3) δ: 1.86-1.90 (m, 2 H), 1.94-1.98 (m, 2 H), 3.42 (t,
J = 6.6 Hz, 2 H), 3.65 (t,
J = 7.0 Hz, 2 H), 7.38-7.41 (m, 3 H), 7.50-7.52 (m, 2 H);
13C NMR
pdf (125 MHz, CDCl
3) δ: 24.6, 26.5, 46.3, 49.7, 127.2, 128.4, 129.9, 137.4, 169.9; HRMS (ESI)
m/z calcd. for C
11H
13NO [M+H]
+ 176.107, found 176.107; MS (EI, 70 eV) 176.0 (66, [M+H]
+), 146 (11, [M+H-C
2H
4]
+), 105 (100, [M+H-C
4H
8N]
+), 77 (45, [Ph]
+); Anal. calcd. for C
11H
13NO: C, 75.40; H, 7.48; N, 7.99; Found: C, 75.08; H, 7.29; N, 7.62.
Handling and Disposal of Hazardous Chemicals
The procedures in this article are intended for use only by persons with prior training in experimental organic chemistry. All hazardous materials should be handled using the standard procedures for work with chemicals described in references such as "Prudent Practices in the Laboratory" (The National Academies Press, Washington, D.C., 2011 www.nap.edu). All chemical waste should be disposed of in accordance with local regulations. For general guidelines for the management of chemical waste, see Chapter 8 of Prudent Practices.
These procedures must be conducted at one's own risk. Organic Syntheses, Inc., its Editors, and its Board of Directors do not warrant or guarantee the safety of individuals using these procedures and hereby disclaim any liability for any injuries or damages claimed to have resulted from or related in any way to the procedures herein.
3. Discussion
The conversion of aldehydes to amides is typically accomplished by two separate steps, i.e. oxidation to a carboxylic acid intermediate and subsequent reaction with an amine in the presence of a coupling agent. Several groups have demonstrated that formation of the amide can be accomplished more efficiently through integration of both reaction steps (oxidation and C-N bond formation) into a single operation. One-pot oxidative aminations of aldehydes can be accomplished with readily available starting materials and this approach avoids the formation and isolation of free carboxylic acid intermediates that often interfere with sensitive functional groups present in the substrate.
Using ammonia and stoichiometric amounts of nickel peroxide, Nakagawa and coworkers were first to demonstrate that aldehydes can be directly transformed into amides.
3 Since then, one-pot oxidative aminations have received increasing attention and several other methods based on the Cannizzaro reaction,
4 the Beckmann rearrangement,
5 and catalytic reactions that require either precious transition metal complexes
6 or
N-heterocyclic carbenes
7 have been reported.
Table 1. Oxidative amination of aromatic aldehydes with secondary amines.
The procedure described herein is most applicable to aromatic aldehydes and secondary amines. It has been successfully used to prepare electron-rich and electron-deficient benzamides derived from pyrrolidine, morpholine or N-methyl benzylamine (see Table for selected examples). While excellent results are generally obtained with aromatic aldehydes, aliphatic substrates give only moderate yields due to competing aldol condensation.
In contrast to previously reported methods, this oxidative amination procedure does not utilize expensive catalysts or additives and proceeds within 6 hours in the presence of readily available tert-butyl hydroperoxide. The mechanism probably involves intermediate formation of a carbinolamine that is oxidized in situ to the corresponding amide while water and tert-butyl alcohol are formed as the side products (Scheme 1).
Scheme 1. Mechanism of the oxidative amination of benzaldehyde to N-benzoyl pyrrolidine using tert-butyl hydroperoxide as oxidant.
Appendix
Chemical Abstracts Nomenclature (Collective Index Number);
(Registry Number)
Benzaldehyde; (100-52-7)
Pyrrolidine; (123-75-1)
N-Benzoyl pyrrolidine: Methanone, phenyl-1-pyrrolidinyl-; (3389-54-6)
tert-Butyl hydroperoxide: Hydroperoxide, 1,1-dimethylethyl; (75-91-2)
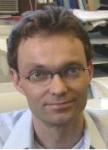 |
Christian Wolf was born in Hamburg, Germany in 1968. He received his Ph.D. under the direction of Professor Wilfried A. König from the University of Hamburg in 1995. After working as a postdoctoral Feodor-Lynen Fellow with Professor William H. Pirkle at the University of Illinois in Urbana he accepted an R&D position at SmithKline Beecham Pharmaceuticals in 1997. In 2000, he began his academic career as Assistant Professor in the Chemistry Department at Georgetown University in Washington, DC where he was promoted to Associate Professor with tenure in 2006. His research interests comprise stereodynamics of chiral compounds, asymmetric synthesis, stereoselective sensing, chiral recognition, transition metal-catalyzed cross-coupling reactions, development of antimalarial drugs, and chiral chromatography. |
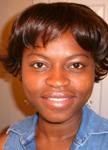 |
Kekeli Ekoue-Kovi Adjoa Sika was born in Lome, Togo. She attended Berea College in Kentucky where she received a B.A. in Chemistry in 2003. In the summer of 2001 and 2002, she gained her first lab experience in David Atwood's laboratory at the University of Kentucky. She is now pursuing a PhD degree in Chemistry under the supervision of Prof. Christian Wolf at Georgetown University in Washington, DC. Her research interests include palladium-phosphinous acid-catalyzed cross-coupling reactions and the development of heme-targeted antimalarial drugs. |
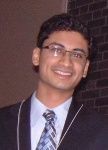 |
Vikram Bhat was born in Ajmer, India. He received his undergraduate education at the Indian Institute of Technology, Bombay, Mumbai. In 2005 he entered the graduate program at the University of Chicago where he joined the research group of Professor Viresh H. Rawal. Currently, his graduate research focuses on the total synthesis of welwitindolinone alkaloids. |
Copyright © 1921-, Organic Syntheses, Inc. All Rights Reserved