Org. Synth. 2007, 84, 102
DOI: 10.15227/orgsyn.084.0102
SYNTHESIS OF ALPHA-HALO ETHERS FROM SYMMETRIC ACETALS AND in situ METHOXYMETHYLATION OF AN ALCOHOL
[1-Methoxymethoxy-1-phenylethane]
Submitted by Martin Berliner
1 and Katherine Belecki.
Checked by William D. Paquette and Peter Wipf
2.
1. Procedure
CAUTION: Chloromethyl methyl ether is an OSHA registered carcinogen and is listed as an extremely hazardous substance by the EPA and the European Community. Other α-halo ethers are likely to be carcinogenic. Exercise due caution to minimize handling of these compounds.
A. Chloromethyl methyl ether as a solution in toluene. A three-necked 500-mL flask is fitted with a magnetic stirbar, thermometer, reflux condenser with nitrogen inlet, and addition funnel (Note 1). The vessel is charged with dimethoxymethane (22.1 mL, 250 mmol, 1 equiv) (Note 2), toluene (66 mL, 3 volumes) (Notes 3, 4) and ZnBr2 (5.6 mg, 0.01 mol%) (Notes 5, 6). The zinc bromide dissolves shortly after addition. Acetyl chloride (17.8 mL, 250 mmol, 1 equiv) (Note 7) is placed in the addition funnel and then introduced into the reaction mixture at a constant rate over 5–15 minutes (Note 8). The addition funnel is rinsed with a minimum volume of toluene (5 mL) directly into the reaction mixture. The reaction self-heats slowly to 40–45 °C and then cools to ambient temperature over 2–3 h, at which time the exchange reaction is typically complete (Note 9). This clear, colorless solution of chloromethyl methyl ether in toluene is employed directly in the subsequent step without further manipulation (Note 10).
B. 1-Methoxymethyl-1-phenylethane. The reaction flask containing a toluene solution of chloromethyl methyl ether (250 mmol, 1.5 equiv) prepared above is immersed in a cold water bath and the contents cooled to 5–10 °C. α-Phenethyl alcohol (20 mL, 166 mmol, 1 equiv) is charged to the addition funnel and added in one portion (Note 11). Diisopropylethylamine (36.0 mL, 208 mmol, 1.25 equiv) is charged to the addition funnel and added to the reaction mixture dropwise over 30 min (Notes 12, 13). The internal temperature of the mixture is maintained below 25 °C during and immediately after the amine addition, and once heat evolution has ceased, the reaction mixture is allowed to come to ambient temperature and then stirred for 12 h. At this time the starting material is consumed (Note 14). The orange solution is diluted with ethyl acetate (100 mL) and a saturated aqueous NH4Cl solution (100 mL), and the biphasic mixture stirred vigorously for a minimum of five minutes to ensure all residual chloromethyl methyl ether is decomposed (Note 15). The layers are separated and the resulting clear, colorless organic layer is washed once with water (100 mL), once with brine (100 mL), dried with MgSO4 and concentrated by rotary evaporation to a clear, colorless oil (Note 16). The material is transferred to a 50-mL round-bottomed flask equipped with a magnetic stirbar and a short-path distillation head. The product is purified by distillation, collecting a single fraction (bp 60–63 °C, 0.5 mmHg) of 1-methoxymethoxy-1-phenylethane as a clear, colorless oil (26.2 g, 157 mmol, 95% yield) (Notes 17, 18, 19).
2. Notes
1.
The success of the reaction does not depend on having previously dried the glassware.
2.
Dimethoxymethane was obtained from Acros Organics at 99.5+% purity and used as received. The submitters used dimethoxymethane, obtained from Aldrich Chemical Co. at 99% purity, as received.
3.
ACS grade toluene was employed without additional purification. A similar grade of dichloromethane, dichloroethane and alkyl acetate esters (i.e. ethyl acetate, isopropyl acetate) can be substituted for toluene with no change in reaction behavior or yield.
4.
Although the reaction can be conducted neat, the authors strongly recommend using a co-solvent to mitigate the strongly exothermic nature of the process. The volume of solvent employed in this reaction was chosen to provide a good balance of reaction rate and thermal control. If less solvent is employed, active cooling should be utilized to keep the reaction temperature below 45 °C.
5.
Zinc bromide (anhydrous powder, 98+%) was obtained from Acros Organics and used as received.
6.
For small- and medium-scale reactions with dimethoxymethane, such small amounts of catalyst are required that the authors found it easiest to dispense the correct amount of catalyst from standard solutions of ZnBr
2 in dimethoxymethane (20–50 mg/mL).
7.
The submitters obtained acetyl chloride from Aldrich Chemical Co. and Acros Organics at 97–99% purity and used it as received.
8.
The reaction temperature increases slightly upon addition of acetyl chloride; the reaction is not dose controlled at this temperature. For a dose-controlled addition, the submitters recommend pre-heating the solvent/acetal/catalyst solution to 40 °C before dropwise addition of the halogenating agent, since the catalyzed exchange reaction is very rapid once the temperature exceeds 40 °C.
9.
A small amount of the reaction mixture (20 μL) is dissolved in 0.6 mL of CDCl
3 for the analysis. The ratio of AcCl (δ 2.6 ppm):MeOAc (δ 2.0 ppm) is utilized to evaluate reaction progress as a small excess of dimethoxymethane is usually present once the exchange is complete (typical ratio MeOAc:AcCl ≥ 99:1).
1H NMR (CDCl
3) δ: 5.44 (s, 2 H, MOMCl), 3.64 (s, 3 H, MeOAc), 3.49 (s, 3 H, MOMCl), 2.03 (s, 3 H, MeOAc).
In situ IR can also be employed to monitor reaction progress without requiring sampling of the reaction contents.
10.
The solution of MOMCl in toluene, prepared using this stoichiometry and solvent volume, has a concentration of approx 2.1 M (18% w/w) with a density of 0.91 g/mL and is stable for months if adequately sealed.
11.
No heat evolution is observed during the addition.
12.
Addition of the amine base results in a strong and extended exotherm as the reaction proceeds. In the absence of external cooling the reaction temperature can exceed 60 °C.
13.
The order of addition of the amine and alcohol can be interchanged without affecting the purity or yield of the product. The following procedure is suggested: The solution of MOMCl is cooled to 10 °C and the base is added, slowly at first, keeping the internal temperature below 25 °C. Alcohol is added in one portion and the solution allowed to warm to ambient temperature and stirred until complete. Workup is as described in the main procedure.
14.
Analysis is by HPLC. Starting material t
R 4.46 min, product t
R 7.25 min. Agilent Zorbax® SB-C8 column 4.6 × 150 mm, 2 mL/min flow, using acetonitrile and 0.1% HClO
4/H
2O as the eluent. The following gradient profile is used: 2 min isocratic (10:90, organic:aqueous), ramp to 90:10 (organic:aqueous) over 6 min, then 2 min isocratic at 90:10 (organic:aqueous). Analysis can also be done by TLC (5:1, hexanes:EtOAc; α-phenethyl alcohol, R
f = 0.13; 1-methoxy-1-phenylethane, R
f = 0.5).
15.
The quench is mildly exothermic as residual MOMCl decomposes. Typically all remaining α-chloro ether has been quenched five minutes after addition of the aqueous solution. Water and saturated carbonate solutions are also effective for quenching the reaction.
16.
Typical mass of crude product is 27 g (98% yield) with purity >95%.
17.
The submitters observed yields from 77–80%. Some decomposition of the product occurs during extended batch distillation, resulting in decreased yields. This is not observed when the purification is conducted on small scale in a Kugelrohr apparatus, or when a chromatographic purification is employed.
18.
The checkers obtained a yield of 90% (12.5 g) on half-scale.
19.
Physical data for the product: R
f 0.5 (5:1, hexanes:EtOAc).
1H NMR
pdf (300 MHz, CDCl
3) δ: 1.49 (d,
J = 6.6 Hz, 1 H), 3.39 (s, 3 H), 4.55 (d,
J = 6.9 Hz, 1 H), 4.59 (d,
J = 6.6 Hz, 1 H), 4.76 (q,
J = 6.6 Hz, 1 H), 7.27–7.37 (m, 5 H).
13C NMR
pdf (75 MHz, CDCl
3) δ: 23.6, 55.3, 73.7, 94.0, 126.3, 127.5, 128.4, 143.2. IR (neat, cm
−1): 2976, 2931, 2887, 1451, 1155, 1099, 1036, 919, 759, 700. Anal. Calcd for C
10H
14O
2: C, 72.26; H, 8.49. Found: C, 72.22; H, 8.64.
Handling and Disposal of Hazardous Chemicals
The procedures in this article are intended for use only by persons with prior training in experimental organic chemistry. All hazardous materials should be handled using the standard procedures for work with chemicals described in references such as "Prudent Practices in the Laboratory" (The National Academies Press, Washington, D.C., 2011 www.nap.edu). All chemical waste should be disposed of in accordance with local regulations. For general guidelines for the management of chemical waste, see Chapter 8 of Prudent Practices.
These procedures must be conducted at one's own risk. Organic Syntheses, Inc., its Editors, and its Board of Directors do not warrant or guarantee the safety of individuals using these procedures and hereby disclaim any liability for any injuries or damages claimed to have resulted from or related in any way to the procedures herein.
3. Discussion
Chloromethyl methyl ether (MOMCl) and other α-halo ethers are versatile reagents for protecting a variety of functional groups
3 and as precursors of carbenes, electrophiles and α-stannyl ethers that have wide use in organic synthesis.
4 This procedure describes an improved method for the generation of α-halo ethers from symmetric aliphatic acetals, including MOMCl and higher substituted analogs.
5 The original preparation of MOMCl in this series
6 and current commercial preparations utilize the reaction between methanol, formaldehyde and hydrogen chloride and produce substantial amounts of the very highly carcinogenic bis(chloromethyl) ether which cannot be readily separated by distillation. More recent procedures
7 have employed the exchange reaction between dimethoxymethane and a halide donor catalyzed by protic acid; these minimize formation of bis(chloromethyl)ether and are preferred to the original preparation. These methods employ different halide sources and different acid catalysts, but all require extended (2–24 hours or more) reflux to effect full exchange, and due to the high vapor pressure of the reactants and products, efficient condensers are required to minimize material loss.
The present method utilizes catalytic zinc(II) salts (0.001 to 0.01 mol%) to accelerate the exchange reaction between halide donors and acetals. Reactions typically are complete in one to four hours irrespective of the scale of the preparation (mmol through mole scale) without the need for external heating. A range of zinc(II) salts can be utilized, with Zn(OTf)2, ZnBr2 and Zn(OAc)2 being the easiest to manipulate. Acid halides and thionyl chloride (but not thionyl bromide) are effective as the halide source (Table 1). A variety of symmetric aliphatic acetals participate in the reaction to give synthetically useful α-halo ethers (Table 2). The solutions of α-halo ethers are employed without isolation in base-mediated reactions where the presence of the ester by-product does not interfere, including alkylations of acids, alcohols, phenols and stabilized enolates under the usual conditions (Table 3). Since α-halo ethers are hazardous (some are carcinogenic; others are possible carcinogens), this procedure illustrates a convenient on-demand synthesis and use of these reagents without isolation or unnecessary handling. The improved safety of this telescoped procedure addresses concerns of both academic and industrial chemists who are under increasing pressure to reduce laboratory hazards.
Table 1. Halogen Sources
Table 2. Acetals Employed in the Exchange Reaction
Table 3: Alkylation Reactions of In Situ Generated α-Halo Ethersa
Appendix
Chemical Abstracts Nomenclature (Collective Index Number);
(Registry Number)
Dimethoxymethane; (109-87-5)
Zinc bromide; (7699-45-8)
Acetyl chloride; (75-36-5)
Chloromethyl methyl ether:
Methane,
chloromethoxy-; (107-30-2)
α-Phenethyl alcohol:
Benzenemethanol,
α-methyl-; (98-85-1)
Diisopropylethylamine:
2-Propanamine,
N-ethyl-N-(1-methylethyl)-: (7087-68-5)
1-Methoxymethoxy-1-phenylethane:
Benzene,
[1-(methoxymethoxy)ethyl]-; (94073-86-6)
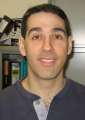 |
Marty Berliner was born in Denver, Colorado in 1968. He received a B.S. in Physics from Harvey Mudd College in 1990 and then switched fields to organic chemistry in graduate school, where he earned a Ph.D. with Tarek Sammakia (University of Colorado, Boulder) developing the asymmetric Diels-Alder reaction of oxocarbenium ions (1996). Following postdoctoral research with David Williams at Indiana University, he joined Pfizer in 1999 and is currently a senior principal scientist in the early candidate group in Chemical Research and Development.
|
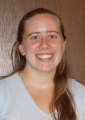 |
Katherine Belecki was born in Silver Spring, Maryland, in 1979. After completing her B.A. in Chemistry at Williams College in 2001, she spent two years working with Dr. Marty Berliner in the early development and discovery support group at Pfizer. She is currently pursuing a doctorate degree in the lab of Dr. Craig Townsend at the Johns Hopkins University in Baltimore, investigating biosynthetic pathways of polyketide natural products.
|
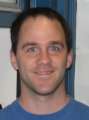 |
William D. Paquette received his B.S. degree at the University of New Hampshire in 2000 and performed research under Prof. Charles Zercher. He then obtained his Ph.D. in 2006 from the University of Notre Dame with Prof. Richard E. Taylor. His graduate research focused on studies toward the total synthesis of 20-deoxyapoptolidin. At Notre Dame, he developed unique methodology to construct contiguous (E)-trisubstituted olefins utilizing a thionyl chloride-induced rearrangement. Currently, he is conducting postdoctoral research under the supervision of Prof. Peter Wipf at the University of Pittsburgh on the synthesis of novel phosphatase inhibitors as well as the natural product wortmannin.
|
Copyright © 1921-, Organic Syntheses, Inc. All Rights Reserved