Org. Synth. 2011, 88, 330-341
DOI: 10.15227/orgsyn.088.0330
SYNTHESIS OF (S)-8a-METHYL-3,4,8,8a-TETRAHYDRO-1,6-(2H,7H)-NAPHTHALENEDIONE VIA N-TOSYL-(Sa)-BINAM-L-PROLINAMIDE ORGANOCATALYSIS
Submitted by Ben Bradshaw
1, Gorka Etxebarria-Jardí
1, Josep Bonjoch
1, Santiago F. Viózquez
2, Gabriela Guillena
2, and Carmen Nájera.
Checked by David Hughes.
3
1. Procedure
A. 2-Methyl-2-(3-oxobutyl)-1,3-cyclohexanedione (1).
A 50-mL Erlenmeyer flask (Notes 1 and 2) equipped with a 4 x 0.8 cm PTFE-coated magnetic stir bar is charged with 2-methyl-1,3-cyclohexanedione (10.1 g, 80.0 mmol, 1 equiv) (Note 3), triethylamine (0.12 mL, 0.80 mmol, 0.01 equiv) and methyl vinyl ketone (6.50 g, 92.7 mmol, 1.16 equiv) (Note 4).
The flask is capped with a rubber septum through which a thermocouple probe is inserted (Note 5), and the heterogeneous mixture is stirred vigorously for 8 h (Note 6).
The initial thick suspension slowly becomes more fluid as the solids dissolve to give a dark brown oil (Note 7).
At the end of reaction, the oil is transferred with EtOAc (100 mL) to a 500-mL round-bottomed flask fitted with a 3-cm oval PTFE-coated stir bar.
Decolorizing charcoal (3 g) (Note 8) is added, and the mixture is warmed to 40-45 °C using a heating mantle and stirred for 30 min.
After cooling to room temperature the mixture is filtered by suction through a 350-mL medium-porosity sintered glass funnel slurry-packed with silica (50 g, 2 cm depth) (Note 9) directly into a 1-L round-bottomed flask.
The silica cake is eluted with EtOAc (4 × 75 mL).
The filtrate is concentrated by rotary evaporation (40 °C bath, 20 mmHg), then dried under vacuum (22 °C, 20 mmHg) for 12 h to constant weight to afford triketone 1 (15.1-15.6 g, 96–99%) as a light yellow oil (Note 10).
B. (S)-8a-Methyl-3,4,8,8a-tetrahydro-1,6-(2H,7H)-naphthalenedione (3). A 50-mL round-bottomed flask fitted with a 2-cm oval PTFE-coated magnetic stir bar is charged with 1 (15.0 g, 76.5 mmol, 1 equiv), catalyst 2 (400 mg, 0.74 mmol, 0.01 equiv) (Note 11) and benzoic acid (230 mg, 1.9 mmol, 0.025 equiv) (Note 12).
The flask is capped with a rubber septum and the mixture is stirred at 22 °C for 5 days (Notes 13 and 14).
After complete reaction, the solution is transferred with EtOAc (100 mL) to a 250-mL round bottomed flask equipped with a 2-cm PTFE-coated magnetic stir bar.
Activated charcoal (3 g) (Note 8) is added, and the mixture is stirred for 15 h at 22 °C.
(Note 15).
The mixture is diluted with hexanes (100 mL) and filtered with suction through a 350-mL medium-porosity sintered glass funnel slurry-packed with silica (60 g, 2 cm depth) (Note 16) directly into a 2-L round-bottomed flask.
The silica cake is eluted with 1:1 EtOAc/hexanes (6 x 100 mL) (Note 17).
The filtrate is concentrated by rotary evaporation (40 °C bath temperature, 20 mmHg) and dried under vacuum (22 °C, 20 mmHg) for 6 h to a constant weight, affording 13.5 g (99% yield) of 3 as a brown oil that crystallizes on standing.
t-Butyl methyl ether (15 mL) is added, and the mixture is warmed to 45 °C with a water bath to dissolve the solids.
The solution is cooled to room temperature over 1 h, then placed in a freezer at -15 °C for 12 h, resulting in the formation of large reddish-brown crystals.
The supernatant is removed by pipette, and the final traces of solvent are removed by vacuum drying to constant weight (22 °C, 20 mmHg, 16 h) to afford (S)-8a-methyl-3,4,8,8a-tetrahydro-1,6-(2H,7H)-naphthalenedione (3) (11.5-11.6 g, 84-85 %) (Notes 18 and 19).
2. Notes
1.
The submitters performed the reaction in a glass vial (3 cm diameter; 10 cm height).
The checkers also carried out the reaction in a glass vial (I-Chem 40-mL borosilicate glass vial, 2.6 cm diameter; 8.5 cm height; equipped with a 2.5 cm octagonal PTFE-coated stir bar).
Due to poor mixing at the initial stages of the reaction, the total reaction time was 1.5- to 2-fold longer than the reaction carried out in the Erlenmeyer flask, but the yield was similar (96%).
2.
No inert atmosphere or special conditions to exclude water were used.
3.
2-Methyl-1,3-cyclohexanedione was purchased from
Sigma-Aldrich (>97%) and
Alfa Aesar (>98%).
Use of the material as received from each source provided the triketone product
1 in >95% yield in 8-12 h reaction time.
The submitters found that on some occasions batches of the impurity-containing starting material had a detrimental effect on the process, not only in the reaction-time to form
1 but also, and most importantly, in the progress of the organocatalyzed formation of
3.
To avoid such problems, the submitters recrystallized 2-methyl-1,3-cyclohexanedione before use.
Material recrystallized as follows reacted in 4-6 h to afford triketone product with similar yield and purity.
To a 1-L round bottom flask fitted with a 3-cm PTFE-coated oval stir bar was added
2-methyl-1,3-cyclohexanedione (25.0 g),
de-ionized water (620 mL) and
absolute ethanol (80 mL, 99.5%, Sigma-Aldrich).
The mixture was heated to reflux (93 °C) over 30 min with stirring using a heating mantle to completely dissolve the solids.
The mixture was allowed to cool to room temperature over 3 h and then stirred at 22 °C for 4 h.
The crystals were collected by filtration, washed with a solution of 7:1
water:EtOH (40 mL), and air dried at room temperature for 12 h to afford 21.5 g (85% recovery) of the dione.
4.
Triethylamine (>99.5%),
methyl vinyl ketone (>99%), and
EtOAc (ACS reagent, 99%) were purchased from Sigma Aldrich and used as received.
5.
The internal temperature was monitored using a J-Kem Gemini digital thermometer with a Teflon-coated T-Type thermocouple probe (12-inch length, 1/8 inch outer diameter, temperature range -200 to +250 °C).
The reaction exothermed to 26 °C during the first hour, then slowly decreased to 23 °C over the course of the reaction.
6.
At the initial stages of the reaction, the mixture was stirred at a speed of 700 rpm.
Erratic stirring occurred due to the presence of solids.
After 15 min, the mixture had become a thick paste and smooth stirring became possible.
After 1 h, the mixture had thinned considerably and the stir rate was reduced to 500 rpm, then to 300 rpm after 3 h.
7.
The reaction was monitored by TLC on silica gel with 1:1 hexanes:EtOAc with KMnO
4 visualization.
The starting material has an
Rf = 0.3; product
Rf = 0.5.
The starting material has a very low solubility in the product oil, so complete reaction is signified by complete dissolution of solids.
8.
Activated carbon (Norit SA3 100 mesh) was purchased from Sigma-Aldrich and was used as received.
9.
Silica gel (50 g, Fisher, 230-400 mesh, 60 Å) was slurried with
EtOAc (100 mL) and poured into the sintered glass funnel that was connected to the round-bottomed flask using a T-type adaptor.
A light vacuum was applied until the EtOAc was absorbed and the silica remained wet and compacted.
The filtration and elution of the product were carried out similarly, with a light vacuum applied and the eluting solution taken down to the top of the silica cake for each wash.
10.
Triketone (
1) has the following physical and spectroscopic properties: IR (film) cm
-1: 1716, 1694;
1H NMR
pdf(500 MHz, CDCl
3) δ: 1.23 (s, 3 H, CH
3), 1.88-1.93 (m, 1 H), 1.98-2.05 (m, 1 H), 2.05 (t, 2 H,
J = 7.4 Hz), 2.09 (s, 3 H, COCH
3), 2.33 (t, 2 H,
J = 7.4 Hz), 2.59-2.73 (m, 4 H);
13C NMR
pdf(125 MHz, CDCl
3) δ: 17.8 (C-5), 20.2 (COCH
3), 29.7 (C-1'), 30.1 (C-4'), 37.9 (C-4, C-6), 38.5 (C-2'), 64.5 (CH
3), 207.6 (C-3'), 210.1 (C-1, C-3).
To obtain an acceptable elemental analysis, the oil was filtered through a 0.45 micron PTFE syringe filter.
Anal.
calcd.
for C
11H
16O
3: C, 67.32; H, 8.22; Found: C, 67.14; H, 7.84.
The material typically contains <1 mol % EtOAc and was >95% pure by NMR analysis.
11.
Catalyst
2 was prepared according to the method described in the preceding procedure.
12.
Benzoic acid (>99.5%),
hexanes (ACS reagent, 99%) and
methyl t-butyl ether (99%) were purchased from Sigma-Aldrich and used as received.
13.
The initial orange solution darkens to black as the reaction progresses.
14.
The progress of the reaction was monitored by taking an aliquot of the reaction mixture and analyzing the C-8a Me peaks by NMR:
1H NMR (400 MHz, CDCl
3); triketone
1: δ 1.23 (s, CH
3), WMK
3: δ 1.44 (s, CH
3).
Following the reaction by TLC is not feasible since the starting material and product have almost identical
Rf values (0.5, EtOAc).
15.
The non-aqueous work up is preferred but affords product contaminated with 0.2-0.3 mol % benzoic acid based on NMR analysis.
An aqueous work up to remove benzoic acid can be carried out as follows.
The reaction mixture is transferred to a 500-mL separatory funnel with
EtOAc (100 mL) and then washed with saturated aq.
sodium bicarbonate (1 x 10 mL) and
brine (10 mL).
The aq.
layer is back extracted with 25 mL of EtOAc.
(The aq.
extractions result in the formation of two black layers such that the phase cut cannot be visibly determined.
Therefore, the aq.
washes are measured and kept to a minimum to facilitate accurate phase cuts.) The combined organic layers are dried over
anhydrous sodium sulfate (30 g) and filtered through a 150-mLmedium-porosity sintered glass funnel into a 500-mL round-bottomed flask.
This solution is taken through the remainder of the workup with activated carbon and silica treatment as described in the body of the procedure.
16.
The silica gel was packed with a 1:1 mixture of EtOAc-hexane and the filtration carried out as described in Note 9.
17.
The filtrate can be collected in smaller flasks and each fraction checked by NMR.
The initial fractions deliver a less-colored product.
However all fractions were found to have similar purity by NMR.
After the indicated washes are completed further washes can be applied and collected in separate flasks to check that no product remains on the silica pad.
18.
(
S)-8a-Methyl-3,4,8,8a-tetrahydro-1,6-(2
H,7
H)-naphthalenedione (
3) exhibits the following physical and spectroscopic properties: reddish-brown crystals, mp 49-50 °C, Lit
450-51 °C;
Rf 0.5 (EtOAc); enantiomeric purity, 96-97% ee; [α]
D25 +93 (
c 1.0
, toluene), Lit
4b: [α]
D25 +97 (
c 1.0, toluene); IR (film) cm
-1: 1714 (C(1)=O), 1667 (C(6)=O), 1620 (C=C);
1H NMR
pdf(400 MHz, CDCl
3) δ: 1.44 (s, 3 H, CH
3), 1.70 (app qt, 1 H,
J = 13.3, 4.4 Hz, H-3), 2.08-2.18 (m, 3 H, H-3 and 2H-8), 2.42-2.53 (m, 4 H, H-2, H-4 and 2H-7), 2.66-2.77 (m, 2 H, H-2 and H-4), 5.84 (s, 1 H, H-5);
13C NMR
pdf(100 MHz, CDCl
3) δ: 23.1 (C-3), 23.5 (CH
3), 29.9 (C-8), 31.9 (C-4), 33.8 (C-7), 37.8 (C-2), 50.8 (C-8a), 126.0 (C-5), 165.9 (C-4a), 198.4 (C-6), 211.1 (C-1); Anal.
calcd.
for C
11H
14O
2: C, 74.13; H, 7.92; O; Found: C, 74.35; H, 7.92.
19.
The checkers determined the enantiomeric purity by chiral SFC: Lux-4 column (150 x 4.6mm, 5um particles); gradient elution, MeOH with 25mM
i-butylamine/CO
2, 4% MeOH hold for 4 min, then ramp at 6%/min to 40% MeOH, hold for 5 min, 15 min total run time; 215 nm detection; 35 °C; 200 bar; (
S)-isomer t
r = 4.1 min; (
R)-isomer: t
r = 4.6 min.
Enantiomeric purity of
3: crude mixture, 90-91% ee; crystallized material, 96-97% ee; supernatant from crystallization, 40% ee.
The submitters determined the enantiomeric composition by HPLC analysis, Chiralcel OD-H column, 96:4 hexane:
i-PrOH, 0.8 ml/min, 254 nm: (
S)-isomer t
r = 23.9 min; (
R)-isomer: t
r = 26.9 min.
Safety and Waste Disposal information
All hazardous materials should be handled and disposed of in accordance with "Prudent Practices in the Laboratory"; National Academy Press; Washington, DC, 1995.
3. Discussion
The Wieland-Miescher ketone (
3)
5 is a useful synthetic building block for which a classical asymmetric procedure using (
S)-proline was published forty years ago.
6Although this method can be applied on a large scale in the laboratory, it has certain drawbacks, partly due to the modest enantiomeric excess (ee) of the product (70%).
To obtain an enantiomerically pure sample from this material, a fractional crystallization procedure
4 allows the undesired enantiomer to be removed by the preferential crystallization of its racemic form, involving the loss of an equal amount of the desired enantiomer.
Moreover, the success of the fractional crystallization has been found to depend strongly upon the ee and chemical purity of the partially enantiomerically enriched form of
3. Thus, although the development of the this procedure enabled a wide application of the enantiopure Wieland-Miescher ketone in natural product synthesis,
7,8 it requires considerable investment of time, material, and experience to achieve satisfactory results and generates considerable waste.
The advent of a plethora of new organocatalysts for aldol reactions has allowed preparation of the Wieland-Miescher ketone (
3) with high enantiomeric excess without
crystallization (86-92% ee),
9 but always conducted on a small scale (0.3-1.0 mmol) due to the use of catalysts that are difficult to prepare (7 to 15 steps) and the requirement for high catalyst loadings (5 to 30 mol %).
Using the method detailed here and the accompanying procedure for the preparation of organocatalyst
2,
10 12 g of the Wieland-Miescher ketone (96-97% ee) can be obtained in 83% overall yield from 2-methylcyclohexane-1,3-dione.
This streamlined procedure
11,12 has significant practical implications from the synthetic point of view since the sequence is performed with high atom efficiency, low catalyst loading (1%), negligible waste formation, and in both steps a short reaction workup and product purification, which enables a large-scale preparation of this bicyclic enedione.
This new methodology for constructing the Wieland-Miescher ketone has broad application in the synthesis of analogs in which the methyl group is replaced by various other alkyl groups that are not easily obtained using proline as the organocatalyst.
Under similar conditions,
11 several triketones undergo cyclization to afford Wieland-Miescher ketone analogs in high yield as shown in Table 1.
Among these, a noteworthy compound for terpene synthesis is the allyl derivative,
13 which is prepared in 93% yield and 97% ee.
Furthermore with both enantiomers of the catalyst readily available the respective WMK enantiomers can be easily accessed.
Table 1. Synthesis of a variety of Wieland Meischer Ketone analogs using N-Tosyl-(Sa)-binam-L-prolinamide as catalyst.
Appendix
Chemical Abstracts Nomenclature (Collective Index Number);
(Registry Number)
(S)-8a-Methyl-3,4,8,8a-tetrahydro-1,6(2H,7H)-naphtalenedione: 1,6(2H,7H)-Naphthalenedione, 3,4,8,8a-tetrahydro-8a-methyl-, (8aS)-; (33878-99-8)
2-Methyl-2-(3-oxobutyl)-1,3-cyclohexanedione: 1,3-Cyclohexanedione, 2-methyl-2-(3-oxobutyl)-; (85073-65-4)
2-Methyl-1,3-cyclohexanedione: 1,3-Cyclohexanedione, 2-methyl-; (1193-55-1)
N-Tosyl-(Sa)-binam-L-prolinamide: 2-Pyrrolidinecarboxamide, N-[(1S)-2'-[[(4-methylphenyl)sulfonyl]amino][1,1'-binaphthalen]-2-yl]-, (2S)-; (933782-38-8)
Methyl vinyl ketone: 3-Buten-2-one; (78-94-4)
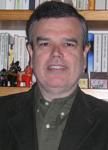 |
Josep Bonjoch was born in Barcelona (Catalonia, Spain) in 1952.
He received his Ph.D.
degree (1979) under the supervision of Prof.
Joan Bosch at the University of Barcelona, Faculty of Chemistry.
He then moved to the Faculty of Pharmacy at the same University, where he was promoted to Associate Professor (1984) and subsequently became Full Professor of Organic Chemistry in 1992.
His main research involves the synthesis of complex nitrogen containing natural products, as a motive for developing new synthetic methodology.
|
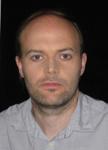 |
Ben Bradshaw was born in 1974 in Southport, England.
He studied Chemistry at the University of Manchester, where he obtained his PhD in 2001 under the supervision of Professor John Joule.
After postdoctoral work with Professor Jim Thomas on the total synthesis of the Bryostatins he joined the group of Professor Josep Bonjoch at the University of Barcelona.
In 2008 he was promoted to the position of assistant professor where his research interests include the application of organocatalysis to the total synthesis of complex natural products.
|
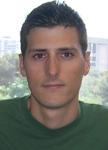 |
Gorka Etxebarria-Jardí was born in 1981 in Barcelona, Catalonia.
He obtained his BSc in Chemistry (2004) and MSc in synthesis of antiretroviral nucleoside drugs (2005) from the University of Barcelona.
In 2006, he joined the research group of Prof.
Josep Bonjoch and is currently completing his Ph.D in asymmetric catalysis and natural product synthesis.
|
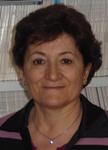 |
Carmen Nájera obtained her B.Sc.(1973) from University of Saragossa and her PhD (1979) at the University of Oviedo under the supervision of J.
Barluenga and M.
Yus.
She performed her postdoctoral work at the ETH (Zurich) with D.
Seebach, at the Dyson Perrins Laboratory (Oxford) with J.
E.
Baldwin, at Harvard University with E.
J.
Corey, and at Uppsala University with J.-E.
Bäckvall.
She became Associate Professor in 1985 at the University of Oviedo and Full Professor in 1993 at the University of Alicante.
Her scientific contributions are focused on synthetic organic chemistry such as sulfone chemistry, new peptide coupling reagents, oxime-derived palladacycles, asymmetric metal catalysis and organocatalysis.
|
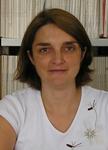 |
Gabriela Guillena received her BSc degree (1993) from University of Alicante.
After spending one year as postgraduate student in the group of D.
Seebach at the ETH (Zurich), she returned to University of Alicante and received her MSc (1995) and PhD (2000) degrees under the supervision of C.
Nájera.
After two years as a postdoctoral fellow at research group of G.
van Koten (University of Utrecht, Netherlands), she returned to the University of Alicante where she became Assistant Professor in 2003 and Associate Professor in 2008.
Her current research interests are focused on new organic methodologies and asymmetric organocatalysis.
|
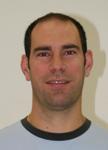 |
Santiago Viózquez was born in Alicante (Spain) in 1981.
He received his B.S.
degree in chemistry at the Universidad de Alicante in 2006.
He is now pursuing his Ph.D.
at the Universidad de Alicante under the supervision of G.
Guillena and C.
Nájera.
His research concerns asymmetric organocatalysis with prolinamides derivatives.
|
Copyright © 1921-, Organic Syntheses, Inc. All Rights Reserved