Org. Synth. 2012, 89, 283-293
DOI: 10.15227/orgsyn.089.0283
Multicomponent Synthesis of Tertiary Diarylmethylamines:
1-((4-Fluorophenyl)(4-methoxyphenyl)methyl)piperidine
Submitted by Erwan Le Gall and Thierry Martens
1.
Checked by David Hughes
1. Procedure
1-((4-Fluorophenyl)(4-methoxyphenyl)methyl)piperidine
1.
To an oven-dried 500-mL, 3-necked, round-bottomed flask equipped with a 4-cm oval PTFE-coated magnetic stir bar is added zinc dust (35 g, 0.54 mol, 9 equiv) and anhydrous acetonitrile (200 mL) (Note 1).
The flask is fitted with a gas inlet adapter connected to a nitrogen line and a gas bubbler.
The other two necks are capped with rubber septa; a thermocouple probe is inserted through one of the septa (Note 2).
To the stirred slurry is added trifluoroacetic acid (1 mL, 13 mmol, 0.2 equiv) and 1,2-dibromoethane (1 mL, 12 mmol, 0.2 equiv).
The stirred mixture (Note 3) is heated with a heating mantle to 50-55°C, held at this temperature for 5 min (Note 4), and then cooled to 25-30°C using a 20 °C water bath.
Maintaining water bath cooling, 4-bromoanisole (33 g, 0.18 mol, 3 equiv) (Note 5) and cobalt bromide (4.0 g, 18 mmol, 0.3 equiv) (Note 6) are added sequentially.
The resulting mixture is stirred for 30 min (Notes (Note 7) and (Note 8)).
Maintaining water bath cooling, piperidine (5.36 g, 63 mmol, 1.05 equiv) is added via syringe in one portion followed 2 min later with 4-fluorobenzaldehyde (7.45 g, 60 mmol, 1.0 equiv) (Note 9), also added via syringe in one portion.
The resulting mixture is stirred for 2 h (Note 10).
The mixture is vacuum-filtered through a 110 mL disposable polyethylene fritted funnel (Note 11) into a 500-mL round-bottomed flask, using t-butyl methyl ether (3 × 50 mL) to rinse the filter cake.
The filtrate is transferred to a 1-L separatory funnel and washed with a saturated ammonium chloride solution (200 mL).
The aqueous layer is extracted with t-butyl methyl ether (200 mL).
The organic extracts are combined and washed with a saturated ammonium chloride solution (200 mL), then vacuum-filtered through a bed of sodium sulfate (50 g) in a 110 mL disposable polyethylene fritted funnel into a 1-L round-bottomed flask, using t-butyl methyl ether (2 × 75 mL) to rinse the filter cake.
The filtrate is concentrated by rotary evaporation (50 °C, 20 mmHg), then held under vacuum (20 mmHg) for 24 h at 60 °C to afford a yellow thick oil containing solids (20-22 g) (Note 12).
This material is purified by alumina chromatography, affording product 1 (16-17 g) that contains 5-7 wt % anisole and 10-12 wt % 4,4'-dimethoxybiphenyl (Notes (Note 13)(Note 14)-(Note 15)).
The highly crystalline biphenyl impurity is removed by crystallization as follows.
To the product in a 500-mL round-bottomed flask is added a 3-cm PTFE coated oval stir bar and hexanes (50 mL).
The slurry is stirred at ambient temperature for 15 min and then filtered through a 40-mL polyethylene fritted funnel into a 250-mL flask, rinsing the filter cake with hexanes (2 × 7 mL).
The filtrate is concentrated by rotary evaporation (50 °C, 20 mmHg), then held under vacuum (20 mmHg) for 24-48 h at 60 °C (to remove anisole), affording 1-((4-fluorophenyl)(4-methoxyphenyl)methyl)piperidine 1 as a pale yellow oil (13.5-14.1 g, 75-78 % yield) (Notes (Note 16) and (Note 17)).
2. Notes
1.
The following reagents and solvents were used as received: zinc dust (Sigma-Aldrich, < 10 μm, ≥98%), acetonitrile (anhydrous, Fisher Optima),
1,2-dibromoethane (Sigma-Aldrich, 99%), trifluoroacetic acid (Fisher certified, 97%), cobalt bromide (Sigma-Aldrich, 99%), 4-fluorobenzaldehyde (Sigma-Aldrich, 99%), piperidine (Sigma-Aldrich, 99.5%),
4-bromoanisole (Acros, 98%, and Sigma-Aldrich, 99%), hexanes (Fisher, ACS reagent, 98.5%),
t-butyl methyl ether (Sigma-Aldrich, >98%), triethylamine (Sigma-Aldrich, 99.5%).
2.
The internal temperature was monitored using a J-Kem Gemini digital thermometer with a Teflon-coated T-Type thermocouple probe (12-inch length, 1/8 inch outer diameter, temperature range -200 to +250 °C).
3.
The magnetic stir bar rotation speed was 500 rpm.
Vigorous stirring is required to suspend Zn dust and the agglomerated zinc suspension after activation.
4.
Gentle evolution of ethylene, resulting from the metallation-elimination of BrCH
2CH
2Br, generally starts between 45 °C and 50 °C, along with the formation of ZnBr
2 which is highly favorable to the forthcoming generation of the organozinc reagent.
Flocculation of the zinc occurs during the activation, constituting a visual confirmation the activation process has initiated.
5.
Three equiv of zinc vs
4-bromoanisole is required to obtain short reaction times.
Lower amounts of zinc leads to incomplete conversion, resulting in contamination of the product with 4-bromoanisole, which is not readily removed by silica or alumina chromatography.
6.
Cobalt bromide is very hygroscopic, so contact with air must be minimized to avoid hydration (the hydration can be observed by the progressive modification of the powder color from intense green to mauve).
7.
Three equiv of
4-bromoanisole vs 4-fluorobenzaldehyde are required to counterbalance the formation of biaryl under these conditions and the loss of 1 equiv of the
in-situ formed organozinc compound upon hydration.
(Further detail on the mechanistic proposal is provided in the Discussion section).
The yield for the formation of the arylzinc species was estimated to be 75-80 % based on quenching the reaction mixture with iodine to form 4-iodoanisole, as follows.
A 0.1 mL aliquot of the reaction mixture was quenched into a solution of
iodine (100 mg) in 2 mL THF.
After standing for 2 min, hexanes (3 mL) and sat.
sodium metabisulfite (3 mL) were added and the vial was shaken to mix the layers.
The organic layer was separated, filtered through a plug of sodium sulfate and then concentrated by rotary evaporation (40 °C bath, 20 mmHg).
The resulting solution was analyzed by
1H NMR, providing the following molar ratio of products: 4-iodoanisole (75-80%), anisole (6-7%, equal to the trifluoroacetic acid charge), 4,4'-dimethoxybiphenyl (7-9 %, equivalent to 14-18 % of the 4-bromoanisole charge).
Diagnostic resonances (CDCl
3, 400 MHz): 4-iodoanisole, δ 3.80; 4-bromoanisole, 3.81, anisole, 3.84; 4,4-dimethoxybiphenyl, δ 3.86.
High levels of residual THF (δ 3.74-3.79) from incomplete evaporation of solvent may interfere with the integration of 4-iodoanisole.
8.
A 20 °C water bath was used to control the exothermic formation of the arylzinc species, maintaining the temperature <40 °C, as profiled in the graph below.
This limited temperature rise is required for complete arylzinc formation.
9.
The reaction temperature increased from 26 to 29 °C on addition of piperidine, then to 36 °C on addition of the aldehyde.
With water bath cooling, the reaction then slowly cools to 23-25 °C over the course of 20 min.
10.
The checker monitored the reaction by
1H NMR by quenching an aliquot of the reaction mixture in 1 mL CDCl
3/1 mL sat NH
4Cl.
The organic layer was filtered through a plug of Na
2SO
4 and then concentrated to dryness.
The residue was dissolved in CDCl
3 for NMR analysis.
Diagnostic peaks: product
1, 4.19 (s, 1H) and 3.77 (s, 3H), 4-bromoanisole (s, 3.80), anisole (s, 3.84), 4,4'-dimethoxybiphenyl (s, 3.87), and 4-fluorobenzaldehyde (s, 9.99).
(The resonances for the methoxy group in these quenching experiments varied slightly from those reported in Note 7 due to differences in sample composition.
Spiking experiments should be carried out for confirmation of individual components).
The submitters monitored the reaction by gas chromatography using a SGE BP1 column, 5 m × 0.25 mm, initial temp 70 °C, ramp at 10 °C/min to 270 °C, hold 15 min.
11.
The polyethylene fritted funnels were obtained from OptiChem.
12.
The oil after rotary evaporation contains about 35 % anisole by weight, which can reduced to <10% prior to chromatography by applying high vacuum (3 mmHg) for 4 h at 60 °C or house vacuum (20 mmHg) at 60 °C for 24 h.
Final weight of the crude material was 20-22 g.
13.
Material was purified by either silica gel or alumina chromatography to remove polar impurities.
Neither method was fully effective at separating the major by-products, anisole and 4,4'-dimethoxybiphenyl.
The best separation of 4,4'-dimethoxybiphenyl was obtained with alumina from E.
Merck (sourced via Acros); however, E.
Merck no longer supplies chromatography-grade alumina.
Chromatography using alumina from either Aldrich (50-150 μm) or Selecto Scientific (N, Super 1, 63-150 μm) is described herein.
A 6-cm glass column was wet-packed with alumina (450 g) using a 95:3.5:1.5 mixture of hexanes:MTBE:Et
3N.
Sand (1 cm) was added to the top of the column.
To the crude resinous product
1 was added 50 mL dichloromethane and 70 g alumina.
The mixture was swirled for 2 min to adsorb thick resinous material on the walls of the flask onto the alumina.
The slurry was concentrated to a powder by rotary evaporation (40 °C, 20 mmHg).
This material was loaded onto the column using
dichloromethane (50 mL).
The column was eluted with a 95:3.5:1.5 hexanes:MTBE:Et
3N mixture (2 L), collecting 50-mL fractions.
Fractions 7-32 were combined and concentrated in potions in a 500-mL round bottomed flask to afford 16-17 g of product
1 containing 5-7% anisole and 10-12 % 4,4'-dimethoxybiphenyl.
14.
The product could also be chromatographed using silica gel (Fisher, 40-63 um, 60 Å) (300 g), wet-packed using a 95:3.5:1.5 mixture of hexanes:MTBE:Et
3N.
The crude product was adsorbed onto silica (50 g) in dichloromethane (50 mL) and loaded on the column.
Elution was carried out with a 95:3.5:1.5 mixture of hexanes:MTBE:Et
3N (1.5 L) then 92:6:2 hexanes:MTBE:Et
3N (1.5 L), collecting 50 mL fractions.
Fractions 7-51 were combined and concentrated to afford 16-17 g of product
1 containing 5-7% anisole and 10-12 % 4,4'-dimethoxybiphenyl.
15.
The checker monitored the chromatography using silica TLC, 90:8:2 mixture of hexanes:MTBE:Et
3N, monitored by UV, R
f anisole = 0.5; product
1 = 0.3; 4,4-dimethoxybiphenyl = 0.3.
Submitters carried out TLC on alumina using a 95.5:3:1.5 mixture of pentane:Et
2O:NEt
3 as eluent, R
f product
1 = 0.65; anisole = 0.90.
16.
Crystallization of
1 was carried out as follows.
Diarylmethylamine
1 (5.0 g) was dissolved in 95:5 EtOH:water (40 mL) at ambient temperature in a 250-mL round bottom flask, then loosely stoppered and placed in a
-20 °C freezer for 18 h.
The resulting while solids were filtered through a 60-mL sintered glass fritted funnel and washed with 95:5 EtOH:water (10 mL, -20 °C), then air-dried at room temperature to afford 3.6 g (72 % recovery) of
1 as a white crystalline solid.
17.
1-((4-Fluorophenyl)(4-methoxyphenyl)methyl)piperidine has the following physical and spectroscopic data: white crystalline solid, mp 38-40 °C; IR, υ (cm
−1) : 2931, 2850, 2789, 2750, 1605, 1504, 1441, 1240, 1208, 1032, 820;
1H NMR
pdf (CDCl
3, 400 MHz) δ: 1.43-1.46 (m, 2 H), 1.53-1.59 (m, 4 H), 2.29 (br s, 4 H), 3.77 (s, 3 H), 4.19 (s, 1 H), 6.80-6.84 (m, 2 H), 6.94-6.98 (m, 2 H), 7.25-7.29 (m, 2 H), 7.33-7.37 (m, 2 H);
13C NMR
pdf (CDCl
3, 100 MHz) δ: 24.9, 26.5, 53.2, 55.4, 75.3, 113.9, 115.3 (d,
J = 21.1 Hz), 129.1, 129.4 (d,
J = 7.9 Hz), 135.2, 139.6 (d,
J = 3.1 Hz), 158.6, 161.8 (d,
J = 244.7 Hz);
19F NMR
pdf (CDCl
3, 376 MHz) δ: -116.35; GC-MS,
m/z (relative intensity): 299 (38) [M
+], 215 (100) [M - C
5H
10N], 204 (19) [M - C
6H
4F], 192 (13) [M - C
6H
4OMe], 171 (35); LC-MS,
m/z (relative intensity): 300.1 (100) [MH
+], 215 (95) [M - C
5H
10N]; Anal.
calcd.
for C
19H
22FNO: C 76.22, H 7.41, N 4.68; found: C 76.16, H 7.80, N 4.68.
GC area % of material prior to crystallization: 97% (t
R = 14.7 min; conditions: Agilent DB35MS column; 30 m × 0.25 mm; initial temp 60 °C, ramp at 20 °C/min to 280 °C, hold 15 min; major impurities 0.5-1.0 % anisole, 0.5-0.6 % 4,4'-dimethoxybiphenyl, 0.1-0.2 % 4-bromoanisole, 0.1-0.2 % 4-fluorobenzaldehyde).
Handling and Disposal of Hazardous Chemicals
The procedures in this article are intended for use only by persons with prior training in experimental organic chemistry. All hazardous materials should be handled using the standard procedures for work with chemicals described in references such as "Prudent Practices in the Laboratory" (The National Academies Press, Washington, D.C., 2011 www.nap.edu). All chemical waste should be disposed of in accordance with local regulations. For general guidelines for the management of chemical waste, see Chapter 8 of Prudent Practices.
These procedures must be conducted at one's own risk. Organic Syntheses, Inc., its Editors, and its Board of Directors do not warrant or guarantee the safety of individuals using these procedures and hereby disclaim any liability for any injuries or damages claimed to have resulted from or related in any way to the procedures herein.
3. Discussion
Diarylmethylamines are compounds of high interest, particularly as active ingredients of several pharmaceutical drugs such as Zyrtec® (cetirizine), Sibelium® (flunarizine), and Iperten® (manidipine) (Figure 1).
While these interesting properties have made them attractive synthetic targets,
2 the synthesis of tertiary diarylmethylamines by one-pot multicomponent procedures
3-4 are rare.
Current methods for the synthesis of these compounds include the Petasis reaction
5 between arylboronic acids, aldehydes, and amines and the aromatic Mannich reaction
6 involving arenes, aldehydes, and amines.
However, despite their important synthetic interest, these reactions present some limitations.
The Petasis reaction requires the use of ortho-activated aldehydes such as salicylaldehydes
5a and the aromatic Mannich reaction is efficient only with electron-rich aromatic compounds.
In addition, in the latter case, control of the carbon that is involved in the C-C bond formation cannot be achieved.
Figure 1. Pharmaceutical drugs containing a diarylmethylamine scaffold
The procedure reported herein describes the synthesis of diarylmethylamines by a one-pot, zinc-mediated, cobalt-catalyzed three-component reaction between aryl bromides, secondary amines and aromatic or heteroaromatic aldehydes.
This method provides convenient access to a range of tertiary diarylmethylamines from common and inexpensive commercial reagents and avoids the limitations of the above-mentioned reactions.
The organozinc reagent, which is the nucleophile involved in this Mannich-like procedure, is pre-formed under conditions similar to those initially described by Gosmini and coworkers.
7 The final compound is obtained through the trapping of an iminium cation (or related species), formed
in situ through the addition of the amine to the aldehyde, by the organozinc species.
A possible reaction mechanism is depicted in Scheme 1.
Scheme 1. Possible reaction mechanism
For efficient preparations of diarylmethylamines by this method, some experimental precautions should be taken: the reaction must be conducted with at least 3 equiv of the organozinc compound to both counterbalance the formation of biaryl, which systematically occurs under these conditions, and furnish an additional equivalent of organozinc compound to efficiently initiate the formation of an iminium equivalent by acid-base reaction.
Zinc dust must also be used in large excess and a limited rise of the medium's temperature must be allowed to ensure a successful formation of the arylzinc species.
The solvent and the catalyst must be anhydrous, otherwise the reaction will require an increased time for completion or not initiate.
This multicomponent procedure is illustrated by the description of a reaction providing 1-((4-fluorophenyl)(4-methoxyphenyl)methyl)piperidine.
However, the scope includes other aldehydes, amines and halides (compounds
2-
16),
8 as depicted in Figure 2.
Figure 2. Some structures accessible by the 3-component coupling procedure
Appendix
Chemical Abstracts Nomenclature (Collective Index Number);
(Registry Number)
Acetonitrile: Methyl cyanide; (75-05-8)
Zinc dust: Zinc; (7440-66-6)
1,2-Dibromoethane: 1,2-Dibromoethane; (106-93-4)
Trifluoroacetic acid: Trifluoroacetic acid; (76-05-1)
4-Bromoanisole: 1-Bromo-4-methoxybenzene; (104-92-7)
4-Fluorobenzaldehyde: 4-Fluorobenzaldehyde; (459-57-4)
Piperidine: Hexahydropyridine; (110-89-4)
Cobalt bromide: Cobalt(II) bromide; (778
9-43-7)
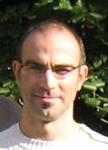 |
Erwan Le Gall received his PhD from the University of Rennes (France) in 1998.
The work was focused on α-aminonitriles synthesis and their applications to the preparation of heterocyclic compounds.
In 1999, he conducted his post-doctoral research at the LECSO group (Paris, France) under the guidance of Dr.
Corinne Gosmini.
He became Assistant Professor (2000) and Associate Professor (2009) at the Université Paris-Est Créteil.
His current research focuses on the development of multicomponent reactions involving preformed or in-situ-generated organometallic reagents. |
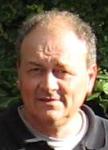 |
Thierry Martens received his PhD from the University Paris 6 (France) in 1988, under the direction of Pr.
Maurice B.
Fleury and Dr.
Martine Largeron.
In 1989, he conducted a post-doctoral work under the guidance of Dr.
Nicole Moreau at the CERCOA CNRS, Thiais.
He joined the Faculty of Pharmaceutical Sciences of the University Paris 5 in 1992 as an Assistant Professor.
In 1994, he joined the group of Pr.
Henri Philippe Husson and of Dr.
Jacques Royer.
His research topic was mainly focused on the development of electrochemical methods for the anodic oxidation of amines.
In 2006, he became full Professor at the Université Paris-Est Créteil.
His current research interests include synthetic electrochemistry and pharmaceutical chemistry. |
Copyright © 1921-, Organic Syntheses, Inc. All Rights Reserved